Conservation of Atlantic salmon - gene banking: principles and considerations
This report examines the procedures, considerations, risks and opportunities of gene banking for Atlantic salmon conservation and restoration in Scotland. Presented is a brief overview of live gene banking and a detailed focus on cryobanking to preserve gametic material through freezing and storage.
Appendix 1. Restoration of wild salmon populations through the use of cryopreserved milt: an illustrative example.
An overview is presented which describes an illustrative example of the restoration of an extirpated salmon population through the use of cryopreserved milt. The scenario is based on a single population where the original stressor has been negated and there is a requirement to restore the stock in regard to fish numbers, while at the same time maximising the reestablishment of the populations natural genetic diversity and associated adaptive capacity.
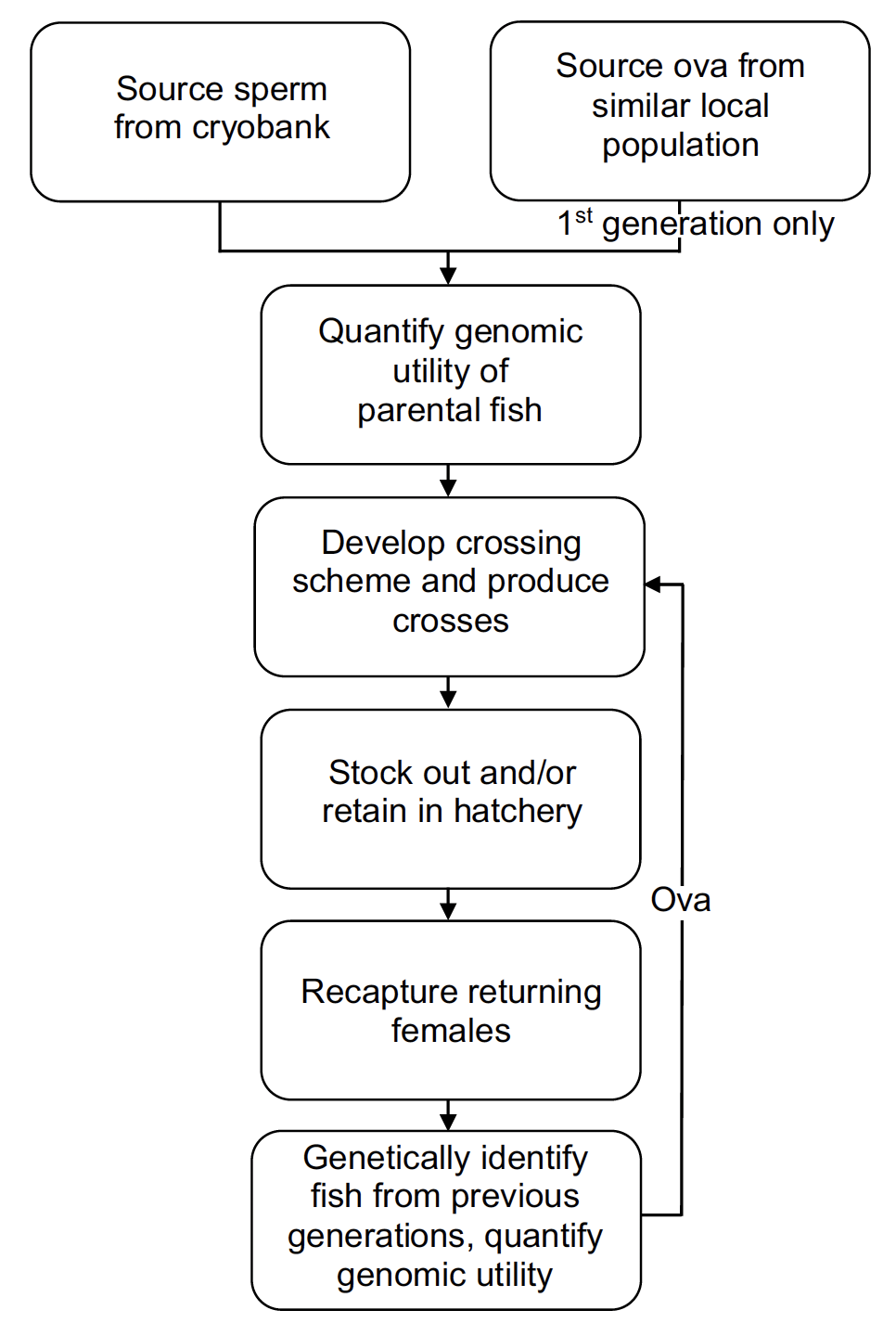
The scenario described here and in the schematic above is one which outlines the various steps required to maximise the potential for positive outcomes and minimise the dangers associated with any artificial restoration scheme. In its most basic form, a restoration project could simply take random sperm and cross it with random female fish from neighbouring rivers and plant out eggs with no regard to the various risk factors such as the use of non-native fish, farm escapes, and inbreeding depression. However, the steps outlined below would ensure that, as much as possible, there would be the maximum beneficial outcome from such a restoration programme while avoiding negative impacts and associated unrealised endpoints.
1) Genetic characterisation and quantification of genomic utility for each male sperm sample
Genetic samples taken from male sperm donors are screened to allow their characterisation in relation to a number of factors. These would include the confirmation that the individual was indeed both native to the population and was not a farmed escapee, to define the relatedness of the individuals, and to identify the proportion of the genome of individuals that is identical by descent (inherited from a common ancestor). In this way only native, wild fish will be used, inbreeding avoided, and the genomic value of all males quantified in regard to their utility for providing maximum genetic diversity in any crossing scheme.
2) Collection of first-generation ova
In the scenario where all native females are lost, ova will be sourced from females from the nearest available wild stocks that are most similar in habitat use and life-history composition. However, considering that there is likely to be spatial coherence in impacts on populations, it may be difficult to remove adult females from neighbouring similarly negatively impacted populations. In such a case, rather than removing adult female spawners to act as broodstock, juveniles and/or smolts could be captured and reared to maturity in a hatchery situation. All females (captured and/or reared) would then be retained in a hatchery until stripping, and as with males, genetically screened to identify farmed escapees, relatedness, and to determine their genomic utility in crossing schemes.
3) Development of crossing scheme
Once the relatedness and genomic utility of the parental fish is known, a crossing scheme would be defined which would minimise inbreeding, maximise genetic diversity, whist at the same time considering any life history phenotype/genotype differences in the original sampled population (e.g. grilse, MSW etc).
4) Stock out
Once crosses have been undertaken, eggs would be stocked out at appropriate densities and in appropriate areas, and/or fish raised to older ages in a hatchery before stocking. In some situations, it may also be the case that fish are reared to maturity for use as broodstock, but in this scenario the risks associated with hatchery domestication would have to be considered.
5) Second and subsequent generation gametes
Creation of first-generation crosses would require the sourcing of ova from females from available nearby stocks until the point when adult females start to return from their marine migrations. At this point ova for the second and subsequent generation crosses would be obtained from adult female fish returning to the river. Again, it is vital that genetic screening is carried out such that farm escapes are identified, only returning native fish are used, and individual genomic utilities quantified. Native returners can be identified using the genetic signatures of the broodstock used in the previous generation crossings (i.e. parental identification / pedigree reconstruction). Sperm could be used from several sources including, of course, the cryopreserved sperm, but also from male returners and precocious parr from previous generations. The use of the cryopreserved sperm would ensure the most rapid dilution of any non-native genome associated with the use of non-native females in the first generation, but other sources would also ensure the maximum genetic diversity was introduced into the new generation. Again, the pedigree and genomic utility of all fish used will be known and detailed crossing schemes developed. This pedigree will grow over time and allow optimal crossing schemes to be produced for every generation to maximise positive realised diversity outcomes.
6) Reestablishment of full native genome
The use of non-native females to produce ova for the first generation will mean that this generation will contain 50% nuclear genomic material from the native origin cryopreserved sperm and 50% from the ova from females from neighbouring rivers. However, this does not necessarily mean that there will be 50% “non-native” genomic material from the ova, as the females will have come from close rivers and the individuals will be related, to an extent, to the native males and so share some degree of genomic material in common. Notwithstanding this observation, and even considering a 50% native / 50% non-native origin, the crossing schemes utilised can, relatively rapidly, dilute the non-native genomic material such that after a relatively few generations the non-native genomic material has all but disappeared.
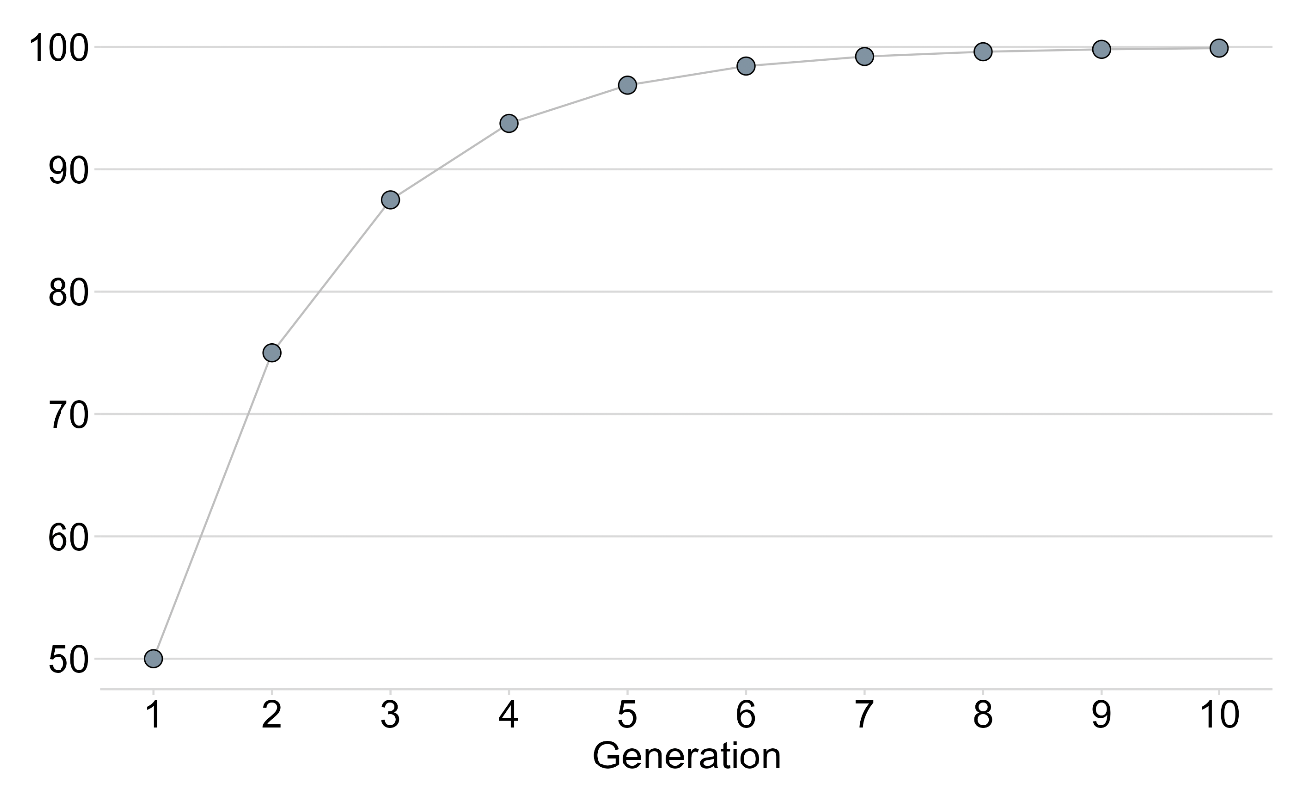
The figure above, which illustrates a simplified scenario, shows that the first generation of crosses would have 50% of genomic material from the non-native females, however, by just the 5th generation the proportion of this material has dropped such that the population is 96.9% native and by the 10th generation 99.9%. In practice, however, as detailed in the main text, there will be distortions and reductions in restoration of the native genome due to absence of mitochondrial DNA in the preserved sperm, effects of hatchery selection, and spawning of F1 and subsequent generations with wild fish.
7) Summary
The above illustrative restoration example describes a situation where the stressor has been removed and restoration is being undertaken, and briefly outlines the various steps required to maximise the genetic restoration of such a population. Careful thought would be required as to how genetic resource preserved from gene banking would be restored as part of the planning process for each specific potential application.
Contact
Email: John.Gilbey@gov.scot
There is a problem
Thanks for your feedback