Offshore wind - diadromous fish: review - January 2024
A report which identifies evidence gaps relating to the potential impacts of offshore wind development on diadromous fish at a strategic level. The report reviews current knowledge and highlights further strategic research opportunities and areas for consideration.
3. Synthesis
3.1 Current information on diadromous fish marine space use
3.1.1 Atlantic salmon (Salmo salar) (SAC qualifying species)
Atlantic salmon is an anadromous species that undertakes long distance migrations between their freshwater spawning grounds and marine feeding grounds. Spawning takes place in the winter and eggs are deposited in gravel. In the UK, juvenile salmon spend 1-4 years in freshwater, until they start their migration to the sea as smolts (known as post-smolts once they exit freshwater and enter marine waters) and head towards the Norwegian Sea. Salmon spend 1-3 years feeding at sea and begin maturing sexually, before returning to their natal stream to spawn (Klemetsen et al., 2003). Although most salmon migrate out to sea, some individuals (almost always males; precocious parr) may stay and sexually mature in freshwater (Myers, 1984; Hutchings & Myers, 1988; Mobley et al., 2021). There is now good evidence that this phenomenon is fairly common and precocious parr can have a significant contribution to the offspring. In fact, in one Spanish population, Saura et al. (2008) estimated that 60% of the offspring paternity could be attributed to mature parr. Bagliniere and Maisse (1985) reported that in two French rivers, occurrence of precocious maturity was <5% in 0+ individuals but in 1+ parr in some years it was as high as 43%. Baum et al. (2004) found that fish size alone was not the main factor for early maturation but instead there was an interaction between size and altitude; for a given size and age, parr in high altitude sites were more likely to be sexually mature.
Salmon smolt migration is seasonal, taking place during the spring in the UK, most often during April and May. However, there are records of populations that have a significant component of autumn migrating individuals, although this feature of the salmon life cycle is understudied (Birnie-Gauvin et al., 2019). In the River Frome (England), approximately 26% of the total downstream migrating individuals migrate in the autumn (Pinder et al., 2007), however these are considered parr at this stage and these fish likely rear and smolt in the tidal regions. The potentially common occurrence of autumn migrating individuals highlights the importance of considering potential impacts on migrating smolts during both spring and autumn.
Repeat spawners should be considered as an important contributor to the dynamics of salmon populations, partly because of their higher spawning potential (Klemetsen et al., 2003). Malcolm et al. (2010) summarised data from five Scottish rivers with 8 to 44 years of data which showed that the mean percentage of repeat spawners varied from 0.71 to 1.48 %. This is a low proportion of the population, compared with observations from other countries. For example, Jonsson and Jonsson (2004) reported that across 17 Norwegian rivers, between 2 and 25 % of salmon were repeat spawners. A more recent review by Persson et al. (2022) found that across 179 Norwegian rivers, the rate of repeat spawners was on average 3.8%, however this ranged from 0% to 26% between rivers.
The migratory life history of Atlantic salmon means that this species may migrate through offshore developments multiple times during their life; as post-smolts, maiden returning adults, kelts and multiple spawners. At all life stages, individuals will be of different sizes, body conditions and have varying prior exposure to developments, therefore it is likely that any potential impact will vary with each life stage.
3.1.1.1 Post-smolts
The feeding grounds of European Atlantic salmon are known to be in the Norwegian Sea and west Greenland (Thorstad et al., 2011). One of the first studies to confirm this was Holm et al. (2000) who conducted nearly one thousand trawls in the Norwegian Sea and Barents Sea for post-smolts. The authors found the highest numbers of post-smolts along the slope current, west of the British Isles and into the Vøring plateau west of Norway. Similar work in the northern Irish Sea and continental shelf area was done as part of the SALSEA-MERGE project (SALSEA-MERGE, 2012). It is important to note however that the results are limited to the specific locations where trawling took place; as the work focused on the slope current, no trawling was done to the west of the current or on the east coast of the UK. Therefore, these studies provide no information on the potential post-smolt movements in those areas. The work by Holm et al. (2000), amongst other previous trawl surveys from several countries, was recently reviewed by Gilbey et al. (2021), which confirmed that the findings of Holm et al. (2000) showed that the highest numbers of post-smolts were found along the slope current and in the Vøring plateau (see Figure 2). Post-smolts are assumed to reach these locations through a combination of active swimming and passive movements following the prevailing current. Recently, studies have found contrasting results (Mork et al., 2012; Moriarty et al., 2016; Ounsley et al., 2020; Newton et al., 2021) and it is likely that the result of the two modes of movement (passive and active) will depend on the location of where smolts first enter the marine environment.
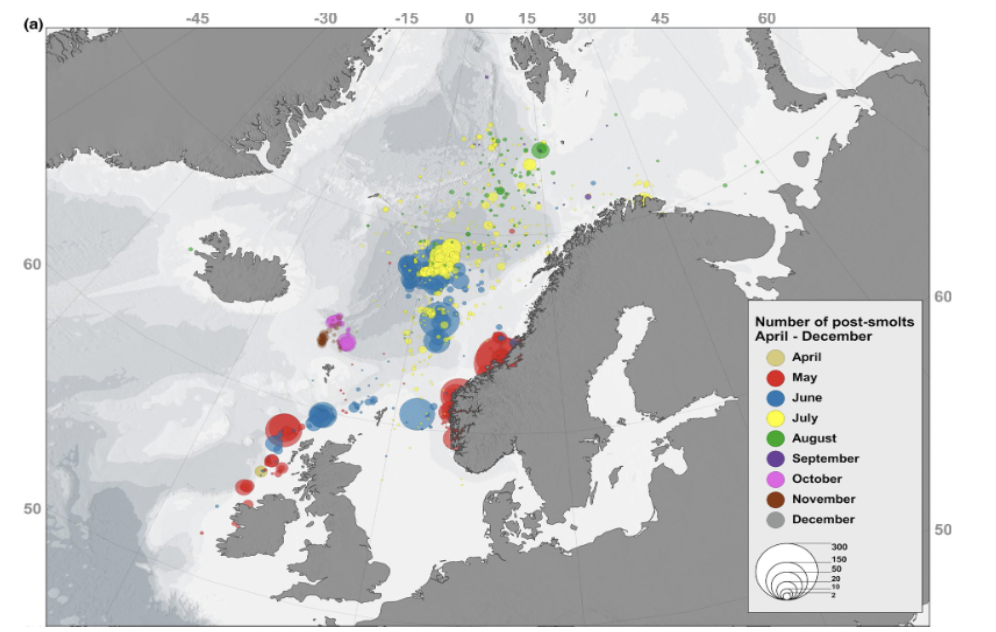
The freshwater component of the salmon migration has been well studied and the location of their marine feeding grounds identified, however, until very recently (2020’s) the pathways used by fish migrating between the freshwater environment and their feeding grounds were not confirmed. There are several large studies (West Coast Tracking Project, COMPASS, SeaMonitor, Derwent Tracking Project; see list of ongoing projects in Appendix) which have recently finished investigating the coastal movement patterns of salmon smolts in the west coast of Scotland (and wider Irish Sea area), but the results of these studies have not yet been published. However, two smaller studies which have been published show the northwards movement of English and Irish salmon smolts, which have the potential to overlap with developments in Scottish waters. In a study of the migration pathways of post-smolts originating from the River Boyne, Barry et al. (2020) detected fish from that river in the Irish Sea (100 individuals tagged, 3 individuals detected at sea), with one smolt detected at an acoustic array located near the Isle of Islay in Scotland. Similarly, Green et al. (2022) showed the marine migration routes of three salmon smolts that had been tagged in the Cumbian River Derwent (a total of 100 individuals were tagged in the river). These smolts were detected moving north near the centre of the Irish Sea, rather than moving near either coastline.
Three studies of the near-shore coastal migration of salmon on the east coast of Scotland have been published (Newton et al. (2021) - the Moray Firth area; Mcilvenny et al. (2021) - northeast near Wick; Main (2021) - near the River Dee). Newton et al. (2021) reported that, upon leaving the River Conon, 56 salmon smolts that were detected at the furthest receiver array did not take the most northernmost route but initially moved on an easterly trajectory. Mcilvenny et al. (2021) reported similar results on Wick River; upon leaving the river, 26 tagged smolts that successfully exited the river did not initially follow a northern trajectory, but instead moved in a direction of a straight easterly line from the mouth of the river. Main (2021) reported the 33 smolts that successfully exited the River Dee, mostly headed southeast rather than migrating north during their first 20 km at sea.
In addition to tracking studies, further work to establish the coastal marine distribution of salmon smolts has been undertaken by Marine Directorate in the form of scientific trawl surveys around the east coast of Scotland. These epipelagic surveys took place in early May in 2017, 2018, 2019 and 2021. The 2017 surveys focused on the Moray Firth area, but the subsequent surveys covered much more of the east coast. Results from these surveys can be seen in Figure 3. It is clear that during May salmon smolts are distributed widely in the seas immediately to the east of the coast (within 150 km from to coast).
In a study by Newton et al. (2021), the swimming depths of salmon post-smolts in the Moray Firth were predominantly located within the top ~2 metres (mean during night: 2.53 m, mean during day: 1.74 m). Work by Davidsen et al. (2008) in Norway reported that salmon post-smolts tagged with acoustic tags with depth sensors were swimming in depths between 0 and 6.5 metres, however during daylight, their swimming depths were mostly between 0 and 3 metres.
Once in the marine environment, salmon post-smolts usually show quick, directed movements towards their feeding grounds. Barry et al. (2020) tracked three salmon post-smolts tagged in Ireland, moving through the same area of the Irish Sea, and these fish had rates of movement of 6-7 km/day. Green et al. (2022) reported rates of movement ranging from 15 to 26 km/day for 3 post-smolts in the same area of the Irish Sea. These differences could be due to population differences, or the varying environmental conditions (mainly water currents) experienced by these fish.
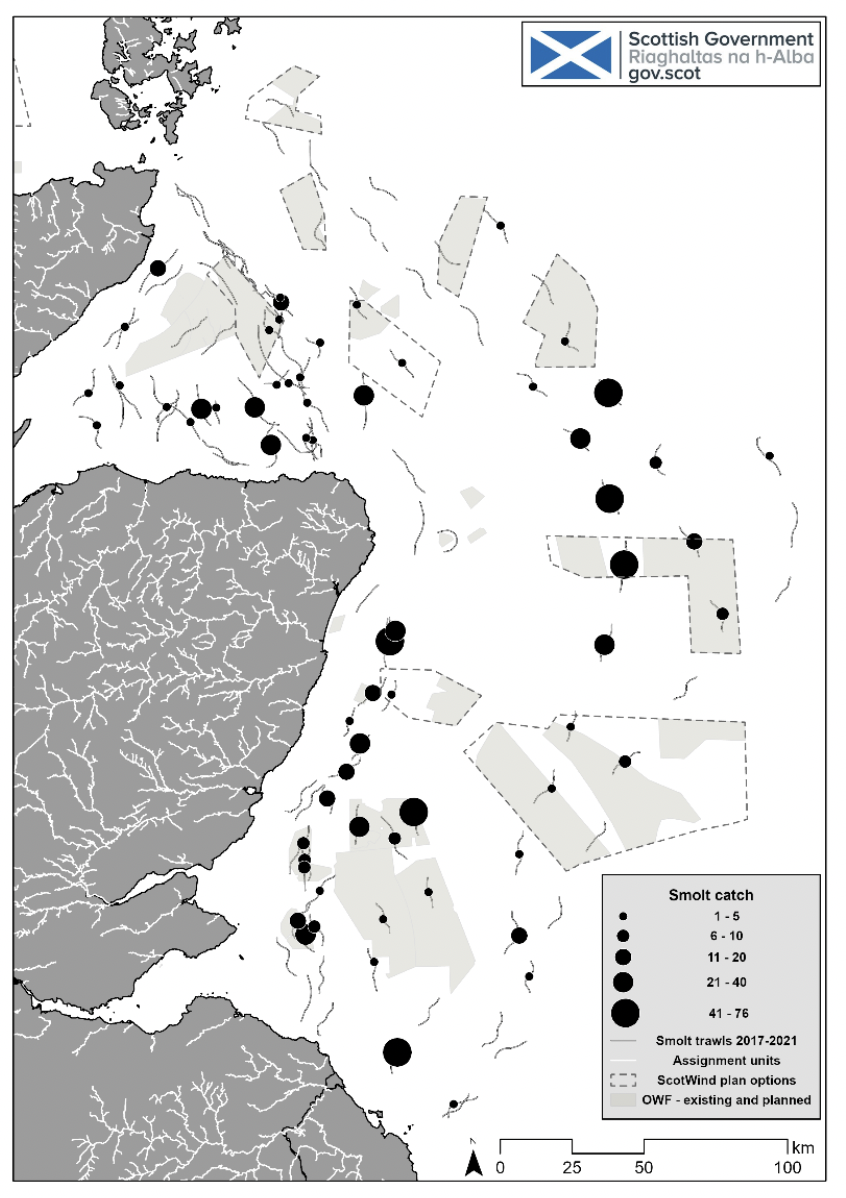
3.1.1.2 Adults (maiden spawners, kelts, multiple spawners)
Over the last decade, sensor satellite tags have been used to gather more information about the exact pathways taken by adult salmon and their migratory behaviour, and in particular their diving behaviour. There is little existing research on the marine migratory pathways for adult salmon in Scotland and the UK. Godfrey et al. (2015) tagged 50 returning adult salmon with satellite tags in northern Scotland. The tags, attached externally to fish captured in a coastal bag net were programmed to release after a short time (1-10 days) and the tag release coordinates were recorded at various locations across northern Scotland (Figure 4). This indicated a range of movements by individuals with some heading further north, while others moved west or east.
Currently, the most detailed information on the movements of Scottish and more widely British adult salmon comes from Carlin tag studies that were undertaken from the 1930’s to 1970’s. These studies have been summarised in detail by Malcolm et al. (2010). One study tagged smolts from the River Tay and its tributaries, between 1967 - 1968. Returning adult fish with Carlin tags were reported from various locations across Scotland, northeast England, and Ireland. This study highlighted that, although the majority of salmon return to their natal streams, at least for some populations, there may be high levels of fish entering a river other than their natal river, although they may or may not spawn there. The results from this study imply that migrating salmon have the potential to interact with multiple offshore developments during their return migrations. Many of the other earlier studies discussed by Malcolm et al. (2010) involved catching returning adults in nets at netting stations, tagging them with Carlin tags and looking for the location of recaptures. These studies were mostly undertaken on the east coast of Scotland but some studies involved tagged salmon from the rivers in the north of Scotland, and showed that salmon were recaptured over very large areas, in some cases up to 500 - 600 km from their tagging site. Several recaptures in these netting stations provide evidence that while adult salmon may move offshore, at least some of them are found along the coastline in the later stages of their return migration to spawn in freshwater. Recaptures to the north and south of tagging locations suggest that adult salmon may approach their spawning rivers from multiple directions, thus using different migratory pathways on their return migration. While these early Carlin tagging studies have provided very interesting data, the studies are limited to point information only and lack the detail of the migration trajectory of the salmon between the first and second capture locations. However as commercial fisheries for salmonids (which provided many of these data) do not exist anymore in the UK, gathering this sort of data nowadays is very limited, highlighting the value of these studies.
There is now considerable evidence that, similar to post-smolts, adult salmon are most often recorded in the top few metres of the water column in the UK and internationally. Godfrey et al. (2015) reported that for returning Scottish adult salmon, 72 - 86 % of the time was spent at depths of 0 - 5 m, and maximum dive depth ranged from 13 to 118 m. In a Norwegian study, Hedger et al. (2022) tagged post-spawning salmon (kelts) with data storage tags to study their migration through a coastal zone and found that the median depths used ranged from 0.3 to 6 m and most dives were shallow (10 - 40 m), although occasional much deeper dives were recorded. Strøm et al. (2018) tagged Norwegian kelts and also reported that more than 83 % of time was spent in the top 10 metres of the water column.
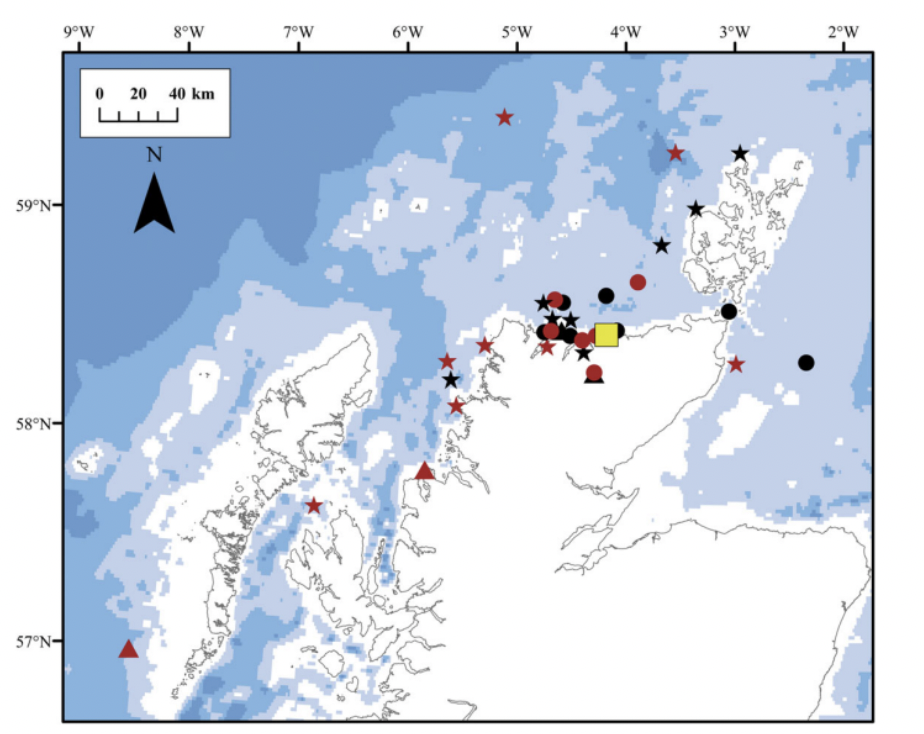
In a large-scale study by Rikardsen et al. (2021), kelts from seven European countries were tagged with satellite tags, enabling their exact migration pathways to be mapped. No UK origin fish were included as part of this study, but fish tagged in southern Ireland migrated along a northeast trajectory, rather than moving north along the continental shelf. Fish tagged from Scandinavian countries were recorded migrating in different directions in the very large area of sea between Greenland and Norway. Similarly, kelts were reported to show high levels of individual variation in migration routes through the Barents Sea in another study by Strøm et al. (2018).
3.1.2 Brown trout (Salmo trutta)
The brown trout is a species that displays partial migration resulting in several different life history strategies (Ferguson et al., 2019). Some individuals are anadromous with a life cycle which is similar to that of Atlantic salmon and are referred to as sea trout. Some trout may only enter estuaries and are referred to as semi-anadromous. In addition to the anadromous life-history strategy exhibited by brown trout, some individuals remain as freshwater residents and complete their whole life cycle in the freshwater environment (potadromous trout) (Klemetsen et al., 2003). This may include remaining in the rivers (river resident) or a migration to a lake. In addition to spring seaward migration, many populations also have autumn migrating smolts, similar to Atlantic salmon (Birnie-Gauvin et al., 2019).
There is good evidence that sea trout are more likely to stray (that is to enter a different stream than their natal stream) compared with salmon (Bekkevold et al., 2020). Bekkevold et al. (2020) used genetic ‘tagging’ to study the origin of net-caught trout in coastal areas of the UK. They found that 34 % of the trout caught originated from a river more than 100 km away from the capture site. The authors also reported a slight preference for southbound migration. Källo et al. (2022) found a 43% straying rate in a Danish catchment. However just because sea trout are found in a non-natal river, it does not mean that they will spawn there; effective straying rate (i.e. proportion of fish that actually spawn and successfully contribute to the next generation in a non-natal river) has been reported to be approximately 1-3% (Jonsson & Jonsson 2006; Ferguson et al., 2016).
Sea trout smolt migration takes place during the spring, in the UK most often during April and May. However, there is evidence that populations have a significant component of autumn migrating individuals, although this feature of the trout life cycle is understudied (Birnie-Gauvin et al., 2019). In Denmark, the proportion of autumn migrant trout smolts has been reported to be as high as 20 - 26% (Winter et al., 2016; Aarestrup et al., 2018; Birnie-Gauvin & Aarestrup, 2019). This highlights the importance of considering potential impacts for migrating smolts not just in the spring but also in the autumn.
Observations have shown that once anadromous brown trout (sea trout) enter the sea, they often remain in the near-shore coastal environment, generally remaining within 80 km of the coast (Thorstad et al., 2016). Nevertheless, there are studies which have shown that some individuals that do undertake long-distance migrations. For example, Kristensen et al. (2019a) reported that some sea trout may make migrations up to 580 km. Additionally, Birnie-Gauvin et al. (2019) highlighted further examples of long-distance marine migrations for sea trout. They noted two Scottish studies; one by Nall (1923) where adult sea trout tagged in the River Tweed in eastern Scotland were later recaptured in southern England, Denmark and the Netherlands, and another by Pratten and Shearer (1983) where smolt and adult sea trout that were tagged on the River North Esk in eastern Scotland were later recaptured in northern Scotland, Norway, Denmark and Sweden. Most of these longer recorded migrations were over 500 km with some up to 800 km in one direction. Many of these older studies relied on capture data from commercial fisheries which no longer exist, and therefore the opportunities for gathering this sort of data are now very limited, giving more value to these studies. Sea trout also usually spend a much shorter period of time at sea compared with salmon, sometimes as little as a few weeks to a few months (Etheridge et al., 2008).
Data from Marine Directorate epipelagic salmon smolt trawls (2017 - 2019 and 2021), show that only relatively small numbers of sea trout have been caught in survey nets. Catches of sea trout have mainly been restricted to the Moray Firth area, although occasional captures have been made further offshore (see Figure 5). Very few individuals have been caught in the trawls closest to the shore. The low numbers of sea trout captured during these surveys may be due to the short duration and timing of this sampling; as the surveys took place in early May each year, targeting salmon post-smolts.
A small number of acoustic telemetry studies on sea trout in Europe and Scandinavia have shown great spatial variability in their habitat usage (Aldvén & Davidsen, 2017). Some individuals migrate out to sea and along the coastline for several hundred kilometres away from their natal rivers (Kristensen et al., 2019b). Flaten et al. (2016) reported that 94 % of post smolts were recorded at least 14 km away from their natal river mouth. There is also evidence that suggests that many sea trout remain in close proximity to their river of origin (Thorstad et al., 2016). Several studies have suggested that anadromous sea trout can also remain in estuaries making greater use of foraging sites there than previously thought (Davidsen et al., 2014; del Villar-Guerra et al., 2014; Aldvén & Davidsen, 2017; Honkanen et al., 2019). Eldøy et al. (2015) demonstrated that veteran migrant sea trout (fish that had spawned at least once before) spent 68 % of their time in a Norwegian marine environment within 4 km of their river of origin. In a Scottish study, Archer (2022) found that trout in the River Dee showed three migration strategies (potadromous, semi-anadromous and anadromous). This study also found that anadromous and semi-anadromous individuals spent more time in the vicinity of the river mouth than the harbour and that the anadromous individuals used littoral, shallow water, and pelagic habitats in equal amounts. Early work on sea trout capture-recapture studies by Nall (1923), Nall (1935), Pratten & Shearer (1983), and Shearer (1990) has been summarised by Malcolm et al. (2010); in these studies, adult sea trout were tagged and their opportunistic capture in coastal nets were recorded. Most of the recaptures were in nearby rivers within approximately 65 km, although a small number of much longer distance migrations further afield in the UK were also recorded. These results were limited by the methodology, as capture in coastal nets and rod fisheries was opportunistic and in limited locations, therefore they provide minimum estimates of migration distances and distribution patterns.
From this body of research, it is thought that there is a dichotomy of spatial range used by sea trout in marine habitats, potentially even within the same population. A study conducted by del Villar-Guerra et al. (2014) demonstrated that there was a split in migratory patterns exhibited by sea trout post-smolts originating from the same river in Denmark, with some individuals remaining in their natal fjord system for over 100 days (53 %), while another subset of individuals migrated out of the fjord within ~40 days (47 %). From this study, it was suggested that once sea trout enter the marine environment, they face a new decision on the adoption of migration strategies; either to remain within their natal fjord system or, to migrate to the open ocean. Ferguson et al. (2019) reported a similar “continuum of migration” for sea trout populations where the extent of marine migration varies from some individuals remaining in coastal estuaries (fish are referred to as “semi-anadromous”) to those that migrate into the open ocean (fish are referred to as “anadromous”).
Despite the studies on the movements of sea trout in the marine environment from elsewhere in Europe and Scandinavia, there is little information on the movements of sea trout in the UK, once they leave their natal river. Observations suggest that the species utilises marine habitats in different ways. For example, it was reported for two Welsh rivers (the Rivers Conwy and Avon) that young sea trout post-smolts move quickly out into the open sea (Moore & Potter, 1994; Moore et al., 1998), mimicking the movements of Atlantic salmon that migrate directly out into deeper water upon leaving freshwater. Pemberton (1976) determined, from extensive seine netting, that Scottish sea trout will move out of their natal fjord-like systems (hereafter referred to by the vernacular term, sea lochs) during the summer in search of food before returning in the autumn.
Other studies have demonstrated that sea trout remain close to their natal river, preferring to forage in coastal sea lochs that provide more estuarine environments. Honkanen et al. (2019) reported that veteran migrant sea trout remained in the inner estuary of a large Scottish sea loch system instead of seeking out deep-water habitats during the summer months. Middlemas et al. (2009) reported that of 48 detected post-smolts in their study, 42 stayed in close proximity to their natal river for the first 14 days after entering the marine environment and ultimately 31 of post-smolts remained within sea lochs less than 6 km from their natal river during the course of the study. Similarly Eldoy et al. (2015) found that 68% of the time tagged Norwegian sea trout were found within 4 km to the river mouths. This possible preference of sea loch habitat by sea trout, particularly young post-smolts (Aldvén & Davidsen, 2017), provides individuals with nutrient-rich environments where the osmoregulatory strain of adjusting to increased salinity is reduced and it is likely that fewer large predators are present (Thorstad et al., 2016).
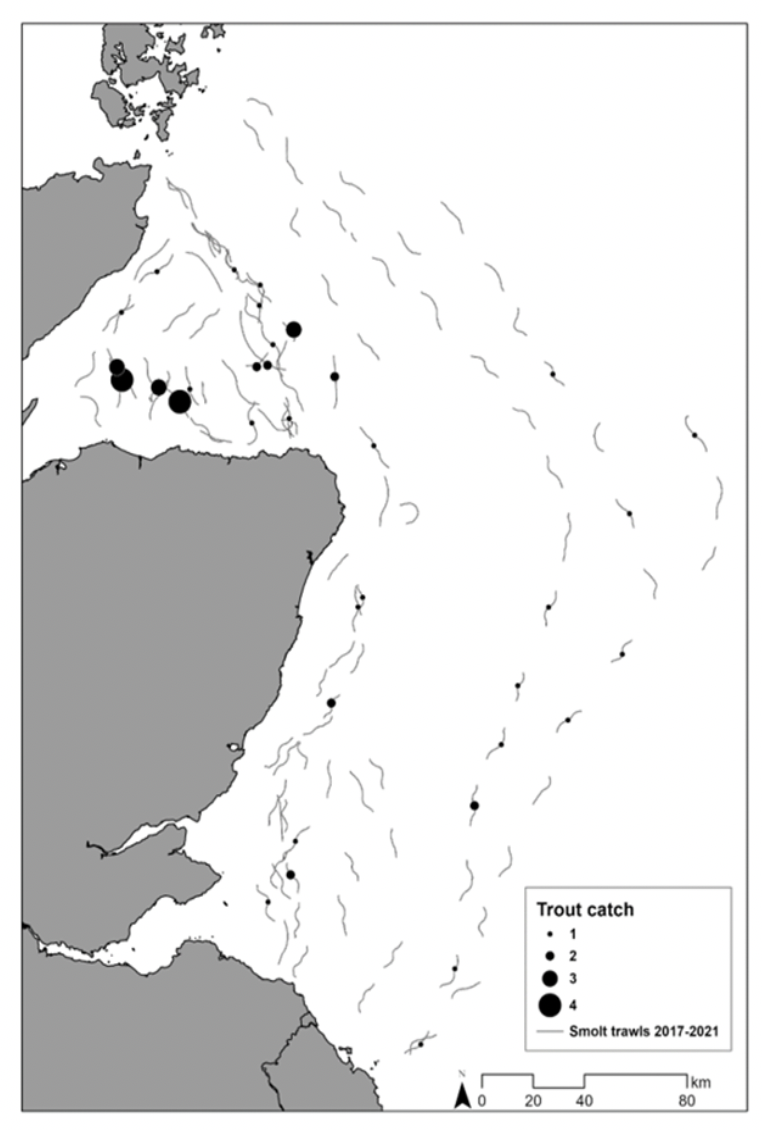
Similar to Atlantic salmon, sea trout have been reported using the top few metres of the water column. Kristensen et al. (2018) tagged Danish sea trout kelts with data storage tags and found that for 64 % of the time, trout were located within the top 0-3 m of the water column. Similar results were also found by Archer (2022) for Scottish sea trout post-smolts; these fish were detected as deep as 8 m but were mostly found in the top 3 meters. In Norway, Eldoy et al. (2017) found that mean swimming depths for sea trout in marine fjord habitats ranged from 0.4 to 6.4 meters. Johnstone et al. (1995) found that post-smolts were mostly found in the top 10 meters, with deeper dives down to 20 meters also observed.
3.1.3 European eel (Anguilla anguilla) (SAC qualifying species)
The European eel is a long-living catadromous species. Recent evidence has confirmed that eels breed in the Sargasso Sea (Wright et al., 2022), where concentrations of very young leptocephali (eel larvae) have been recorded. Following spawning, the leptocephali move across the Atlantic Ocean with the help of ocean currents and, after reaching the continental slope in Europe, they metamorphose into glass eels. Eels develop and grow in freshwater and migrate seaward as partially sexually mature adults after 5 – 25 years (van Ginneken & Maes, 2005; Cresci, 2020). Downstream seaward migration most often takes place in the autumn. European eel are semelparous (which means that they spawn once in their lifetime and all adults die after spawning). European eels have a complex life cycle with several stages, but this section focuses on the migratory life stages that are most likely to overlap with offshore developments.
3.1.3.1 Larval migration
The migration of leptocephali and the next life stage, glass eels, is not well understood, as it is currently not possible, due to size constraints, to use telemetry to track eels at these life stages. The available data comes from captures of individuals at sea and using their lengths to determine possible pathways along a growth gradient; much of this work was done by Johannes Schmidt in the early 20th century using fine meshed plankton nets. In surveys, leptocephali <12mm have been found across a longitudinal range of 2000 km between 50 and 70 degrees north (Miller et al., 2019). The leptocephali are thought to be unable to swim actively and, therefore, simply drift with oceanographic currents towards and onto the continental shelf. Thus, their movement speeds will be determined by the current speed. As they turn into glass eels, they develop the ability to swim - work by Naisbett-Jones et al. (2017) has shown that this life stage is able to detect changes in the Earth’s magnetic field to orient. As they reach coastal waters, the influence of near shore coastal currents has been linked with their recruitment to fresh waters as glass eels (Barry et al., 2015). Based on data from Schmidt and a few later studies (e.g. Miller et al., 2019), it has been indicated that larval and post-larval stages (leptocephali and glass eels) migrate from the Sargasso Sea towards the UK using oceanic currents and, therefore, current patterns can be used to estimate the potential migration routes of juvenile eels. The depth zone used by leptocephali and glass eels is not well known, however, Taning et al. (1938) sampled leptocephali (18 - 50 mm length) near Bermuda and caught them predominantly at depths 45 - 365 metres but deeper observations were also reported.
3.1.3.2 Adult migration
Righton et al. (2016) used satellite tags to track the oceanic migration of 87 adult European eels from four study areas (the Baltic Sea, Celtic Sea, North Sea and the Mediterranean; see Figure 6) towards their migration area at Sargasso Sea. While the satellite tags provided a range of data over varying distances, many of the tagged eels seemed to be heading to the direction of the Azores. The last segment of the migration route was confirmed by Wright et al. (2022) who satellite tagged and tracked the movements of 21 adult European eel in the Azores, demonstrating that the eels migrate towards the Sargasso Sea along the Mid-Atlantic Ridge.
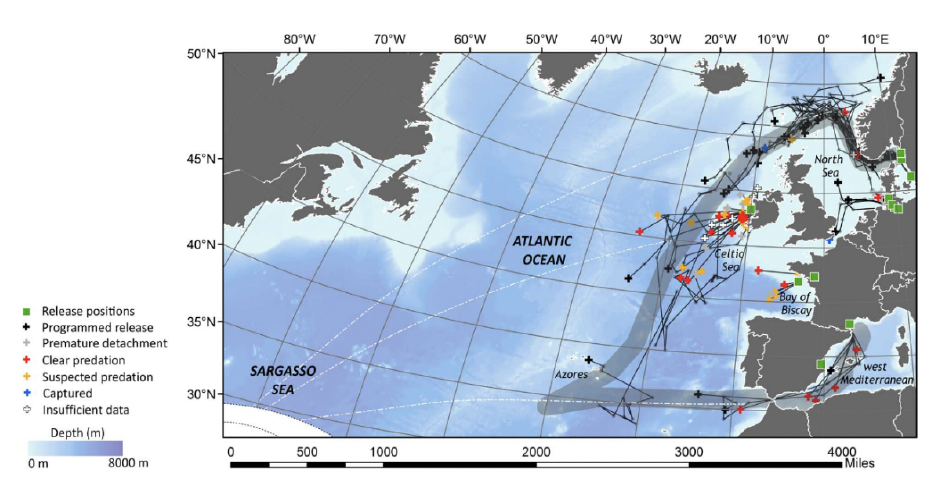
Righton et al. (2016) showed that eels migrating back to their presumed spawning grounds (silver eels) from Scandinavian and possibly German populations migrate past northern Scotland on their way to the Sargasso Sea, highlighting that offshore renewable developments in Scottish waters could potentially impact populations from other countries. Righton et al. (2016) did not tag any eels from the United Kingdom and therefore there is no direct evidence of the coastal migration pathways taken by eels from UK populations. However, considering the migration route taken by the Scandinavian populations and some German populations, it could be inferred that eels from the east coast of Scotland may initially move north before heading south-west towards Sargasso Sea. Eels from populations from the west coast of Scotland may head directly west or south-west to join the migration pathway of Scandinavian and Irish populations.
An acoustic tagging study from the River Foyle in Ireland (Barry et al., 2016) recorded swimming speeds of 0⋅006 – 0⋅040 m/s equating to a total daily distance travelled of between 0.05 to 3.48 km in Lough Foyle which is a brackish sea lough. Aarestrup et al., (2009) used satellite tags to track silver eels from Ireland and found that the net migration speed at sea was 13.8 km/day (ranging from 5 to 25 km/day); however, the authors note that this speed would not be fast enough to reach the Sargasso Sea in time for the spawning season. Wright et al. (2022) used satellite tags to track adult eel from the Azores and noted migration speeds between 3 - 12 km/day. Adult eels swim in deep waters and appear to show distinct diurnal diving behaviour; Aarestrup et al. (2009) reported that during the day, eels were found in deep, cool waters at an average depth of 564 m and during the night, the eels moved much up to much shallower depths but still remained at an average depth of 282 m.
3.1.4 Three-spined stickleback (Gasterosteus aculeatus)
Three-spined stickleback (hereafter stickleback) show significant variation in their migratory patterns; they are often considered a freshwater species but can also be anadromous. It is thought anadromy is the ancestral ecotype and freshwater residency has since evolved multiple times (Haglund et al., 1992). Spawning migration to rivers and estuaries usually occurs in the spring and juveniles migrate to sea in the autumn, however there is considerable inter-population variation in the migration timings (Kitano et al., 2012). Freshwater and anadromous populations can interbreed but are usually reproductively isolated (Hagen 1967, Hagen 1973).
Information relating to three-spined stickleback’s freshwater ecology is well documented from laboratory and field studies. There is, however, less information available, especially in the UK, about their diadromous life history. Some studies have reported observations of offshore anadromous stickleback. Quinn and Light (1989) caught stickleback in the north Pacific Ocean as bycatch; 1.86 % of their purse seine sets included stickleback, the most distant observation, 945 km from land. Williams and Delbeek (1989) collected stickleback in the Bay of Fundy (Canada), with some individuals being recorded 50 - 100 km from land.
In the UK, there are many records of three-spined stickleback in estuaries. A 5-year study by Claridge et al. (1986) in the Severn estuary reported that sticklebacks were commonly found in several coastal power station inflows, indicating their presence in the coastal environment. Individuals were most commonly recorded in the inner estuary but occasionally also further out in the inner channel. Jones (2005) reported an anadromous population in the Scottish River Tyne. Stickleback have also been found on the tidal sands of Culbin Sands in the Moray Firth (Mendonca, 1997), in the Forth estuary (Elliott et al., 1990) and Loch Etive (Carss & Elston, 2003). Araujo et al. (1999) recorded stickleback in the upper Thames estuary.
Records of three-spined stickleback in the open ocean waters surrounding the UK are rarer. One of the earlier records by Jones and John (1978) reported the capture of one live stickleback in the north Atlantic (59°N, 19°W), 400 miles from the nearest landmass. The authors also reported evidence of cod feeding on sticklebacks. Hislop (1979) reported stickleback from trawl sampling in the North Sea, north of Scotland. The authors conducted two survey trips during the autumn in 1977 and 1978, mostly catching a few stickleback with each haul but in 1978 across two sites they caught 129 stickleback, suggesting they might be shoaling in the marine environment (Figure 7). The Marine Directorate conducted epipelagic trawl surveys in and around the Moray Firth area in eastern Scotland, targeting salmonid smolts. As part of these surveys, sticklebacks were a common bycatch species (Figure 8). Large numbers (up to 350) per trawl were caught in trawls within the Moray Firth, but smaller numbers of individuals were also caught over 150 km from the shore. Additionally marine stickleback were recorded in large numbers within 16 km from the coast of the Isle of Man (Bruce et al., 1963).
Reports suggest that stickleback use the very top of the water column when at sea. Williams and Delbeek (1989), in addition to Hislop (1979), caught stickleback by trawling the top 1 metre of the water column. The Marine Directorate smolt trawl surveys were targeting the top 12 meters of the water column.
There are no studies available within the literature which can provide estimates of the actual migration speeds of anadromous stickleback, however Taylor and McPhail (1986) found in a laboratory trial that anadromous individuals can maintain prolonged swimming speeds of 5 body lengths/s for much longer periods than resident individuals (although residents can achieve higher burst swimming speeds).
While the studies above confirm the presence of three-spined stickleback in oceanic waters, including in UK waters, there is no evidence available to confirm migration pathways or the originating freshwater population of marine sticklebacks.
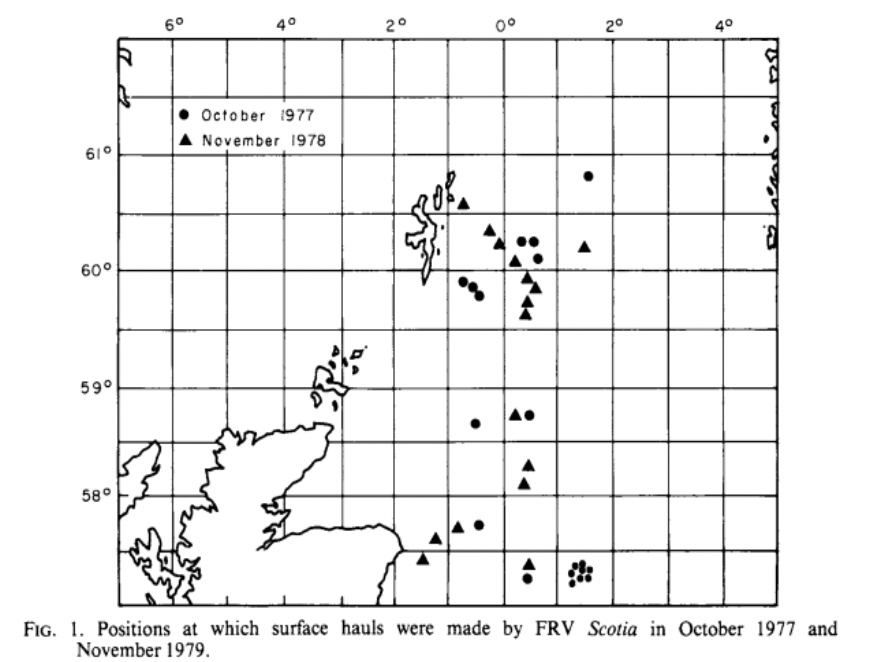
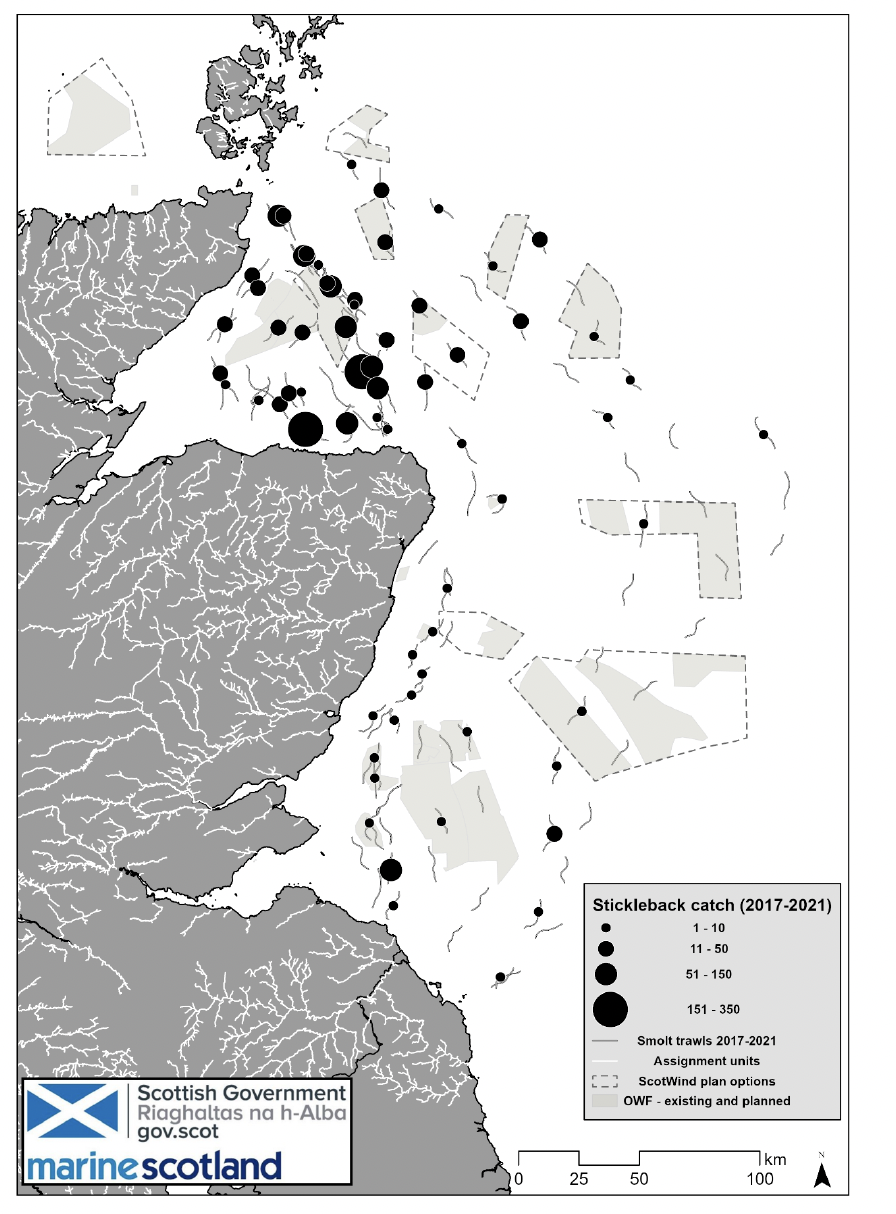
3.1.5 River lamprey (Lampetra fluviatilis) (SAC qualifying species)
River lamprey spawning takes place in rivers and eggs are laid in simple nests or shallow depressions in stony or gravelly stretches with good water flow. After a few weeks, the larvae (ammocoetes) hatch and move (either by swimming or carried by the flow) to areas with fine sediment. They remain in these larval habitats for 3 to 8 years and then undergo a metamorphosis into a parasitic life cycle stage. Once metamorphosed, river lamprey migrate downstream (known now as ‘transformers’) and to the marine environment where these pre-adults feed parasitically on other fish. River lamprey have been reported to prey on multiple species, including salmonids and gadoids (Quintella et al., 2021). After 3 - 24 months, the adults return to freshwater to spawn (Kelly & King, 2001; Maitland, 2003; Elliot et al., 2021).
River lamprey seaward migration usually does not extend to coastal waters, with individuals remaining in estuaries. While the freshwater life stage is very well studied, there is very little information available on the spatial distribution of river lamprey once they have left freshwater. It is known that river lamprey require good water quality in freshwater and estuaries. In river systems such as the Clyde, where water quality used to be very low, populations disappeared (Maitland, 2003). Maitland et al. (1984) provide some information on the timing of migrations. The authors studied river lamprey caught in the intake screens of power stations in the estuarine waters of the Firth of Forth and reported that downstream migration took place in the spring (individuals size ranged from 69 - 135mm), while sexually maturing adults (200 - 361mm) were caught in the late summer and autumn. This would suggest that, at least at this location, estuarine feeding was most likely a few months in duration. In a similar study in the Severn estuary, Abou-Seedo and Potter (1979) reported that the number of upstream migrating adult river lamprey started to increase in October and November, a few months later than the Maitland et al. (1984) study. Abou-Seedo and Potter (1979) hypothesised that the increased discharge rate from the river was a key factor initiating upstream movement.
Little is known about the downstream migration and marine life of river lampreys (Lucas et al., 2021). The best information on this comes from Elliot et al. (2021), who collated data from fisheries-dependent and fisheries-independent surveys between 1965 and 2019 within the Greater North Sea, Celtic Sea, Bay of Biscay, Iberian Coast and Metropolitan French waters. The presence of river lamprey as bycatch was noted; and only 300 river lamprey were recorded in over 168 000 hauls. Most river lamprey were caught by bottom trawl surveys and demersal gear, suggesting that river lamprey are swimming in deep waters. River lamprey were mainly caught in the southern North Sea near Dutch and German coasts, but some individuals were caught near south west England (Figure 9; purple icons). The length of river lamprey captured varied from 14 to 42 cm, with a positive relationship noted between length and distance from shore. However, it was not possible to identify where captured individuals originated from in this study. More recent work by Elliott et al. (2023) using a similar data set shows similar patterns with river lamprey bycatch reported in south-east England (see Figure 10). Despite many trawls along the east coast of northern England and southern Scotland, no river lamprey were reported in the catches. However, it should be noted that the different gear types used in the data set are likely to significantly alter the catchability of specific species and therefore this data set may not be fully representative of the real distribution.
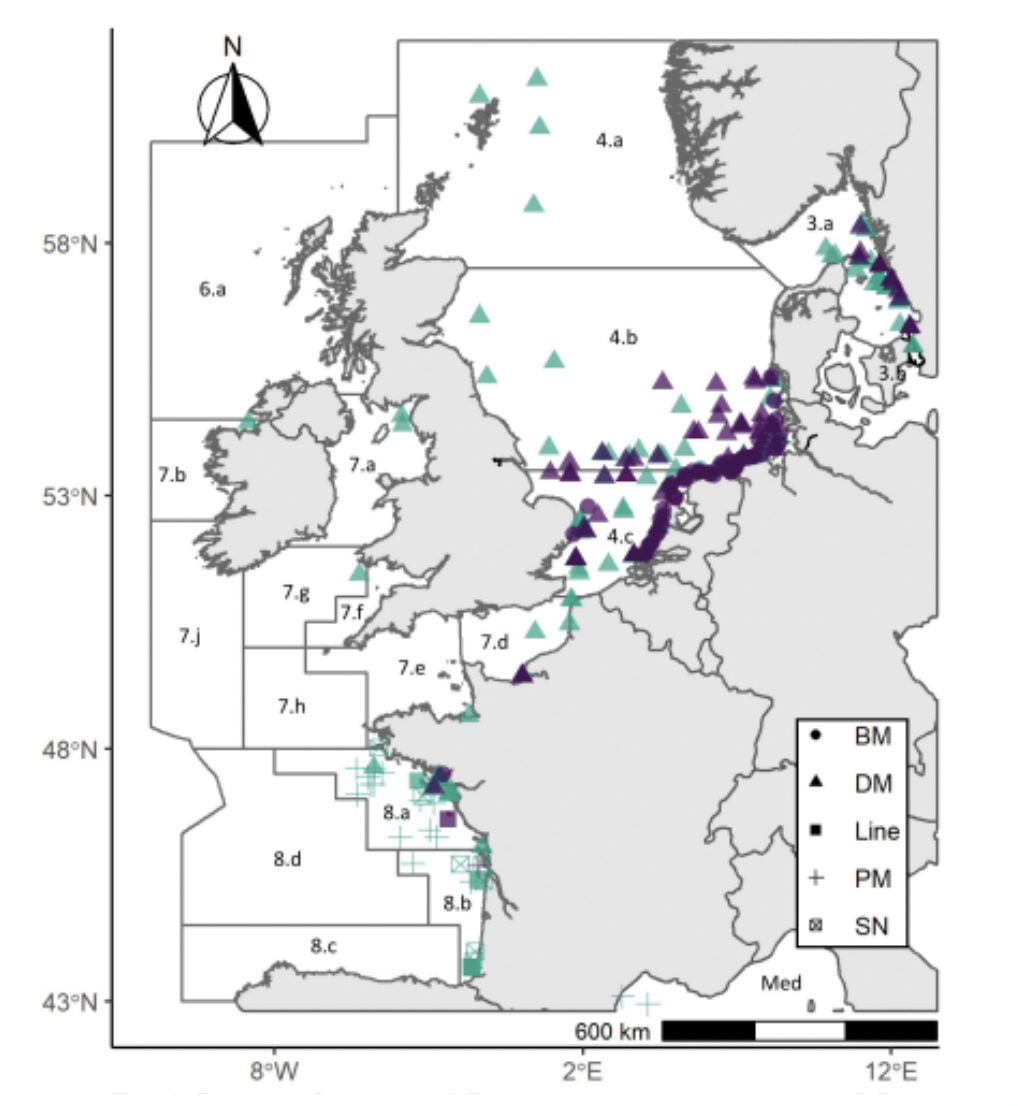
No tracking studies that focus on marine movements and habitat use of river lampreys in the UK or Europe have been identified as part of this review. Therefore, it is not possible to determine the spatial extent of these migrations beyond the opportunistic trawling studies such as Elliott et al. (2021 & 2023). There is very little data to show the presence of river lamprey outwith estuaries in the UK waters, however there is some evidence of river lamprey being found further offshore in continental Europe and therefore it is possible that they may come into contact with offshore renewables.
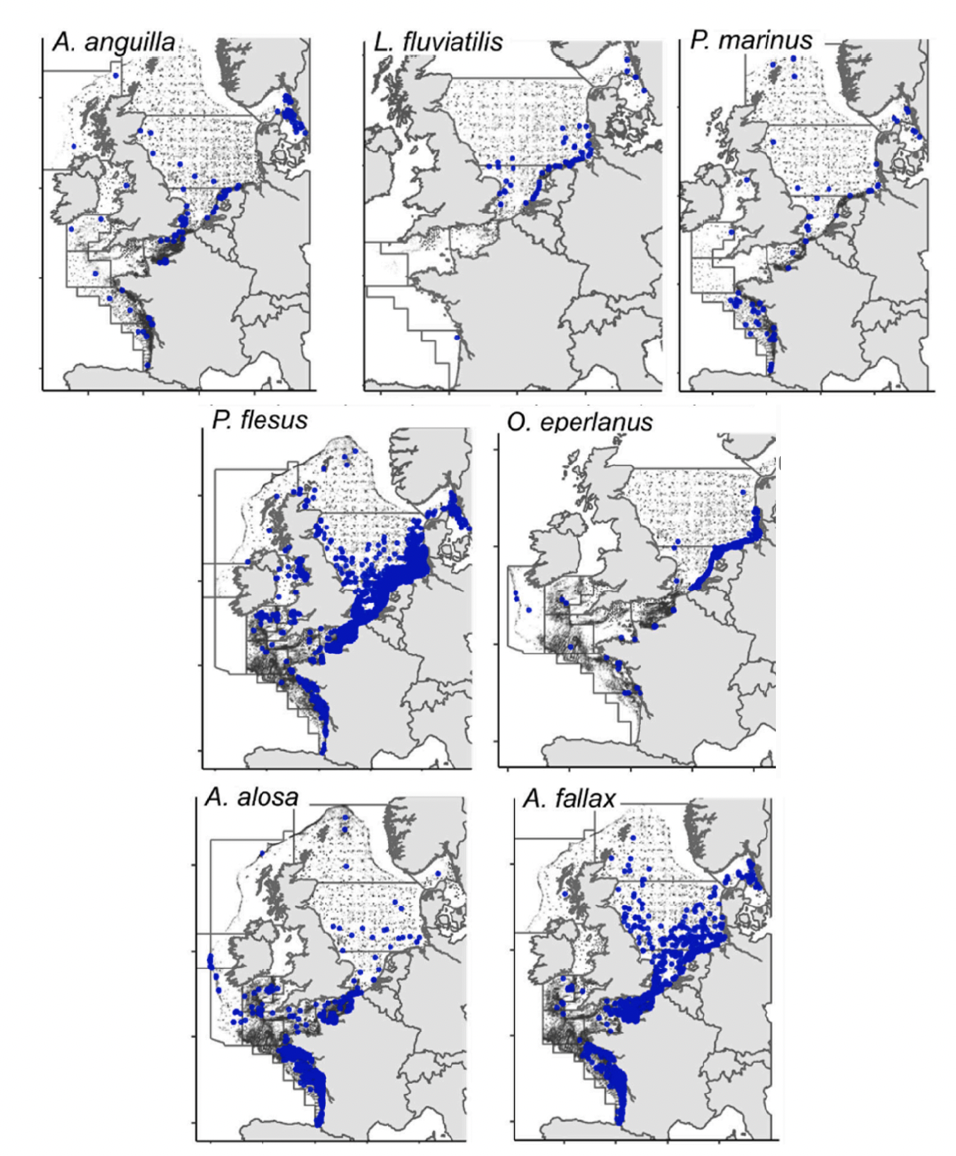
3.1.6 Sea lamprey (Petromyzon marinus) (SAC qualifying species)
The sea lamprey life cycle is similar to that of river lamprey; they spawn in the freshwater in gravelly areas with good water flow. After hatching, larvae emerge and swim or drift downstream where they search for silt beds in which to burrow. This larval stage lasts several years but there is significant variation (Kelly & King, 2001; Hansen et al., 2016). After a period of larval growth, sea lamprey metamorphose over a period of a few weeks, to the parasitic life stage. These migrate downstream to sea to feed parasitically on fish. Sea lamprey have been reported feeding on a large variety of fish species (Quintella et al., 2021). Spawning migration upstream usually takes place from April onwards and appears to be initiated by temperature (Maitland, 2003).
Sea lamprey have been found in shallow coastal areas and deeper offshore waters (Maitland, 2003). However, very little information is available for the marine life stage of sea lampreys (Lucas et al., 2021). The best information on spatial distribution comes from Elliot et al. (2021), who collated data from fisheries-dependent and fisheries-independent surveys between 1965 and 2019 within Greater North Sea, Celtic Sea, Bay of Biscay, Iberian Coast and Metropolitan French waters. The presence of sea lamprey as bycatch was noted; only 421 sea lamprey were recorded in over 168 000 hauls. Most sea lamprey were caught by bottom trawl surveys and demersal gear, suggesting that sea lamprey are swimming close to the bottom. There are records of sea lamprey caught at various points in the North Sea from north of the Shetland Islands to southern France (see Figure 8; green icons). However, as part of this study, it was not possible to identify from where the captured individuals originated. The size of captured sea lamprey varied from 13 to 92 cm, and there was a positive relationship between size and distance from the shore. More recent work by Elliott et al. (2023) reported captures of sea lamprey to the east of Scotland (Figure 10). However, it should be noted that the different gear types used in the data set are likely to significantly alter the catchability of specific species and therefore this data set may not be fully representative of the real distribution.
No tracking studies that focus on marine movements and habitat use of sea lampreys in the UK or Europe have been identified as part of this review. It is not possible to determine the spatial extent of these migrations beyond data provided by Elliott et al. (2021).
3.1.7 European flounder (Platichthys flesus)
European flounder is typically found in marine and brackish waters but is also frequently found in freshwater. It has a near ubiquitous distribution in UK waters and is found around all of the mainland coastline and most islands. The flounder is catadromous with breeding occurring in coastal waters from February to June. However, there is some evidence of flounder also being able to spawn in brackish estuary waters (i.e. in Portugal, Morais et al., 2011). Flounder is a broadcast spawner. Juveniles are initially symmetrical and metamorphose into the flatfish form when they are around 10 - 15 mm long and will start migrating towards estuaries before metamorphosis. Males reach sexual maturity before females.
Flounder typically show daily migration with the tides, moving into intertidal zones with the rising tide to access good quality feeding grounds (Raffaelli et al., 1990). Flounder are commonly found within 50 km from the shore (Skerrit, 2010). On a greater temporal scale, flatfish move between breeding and feeding grounds. Therefore, offshore developments in the coastal zones are likely to have different potential impacts (Barbut et al., 2020).
Quite likely due to the difficulty of tracking marine species, there are still significant knowledge gaps relating to the movements of flounder, especially for juveniles (Le Pape & Cognez, 2016). It is possible to tag flounder with acoustic tags, however external tagging (rather than internal tagging) should be used. Neves et al., 2017 found that fish subject to internal tagging had very low survival rate (10 %) while external tagging did not seem to affect their behaviour (although there was a negative effect on condition). The potential negative impact of tagging is something that should be considered as part of every tagging study.
Outside daily feeding and seasonal breeding migrations, flounder appear to exhibit high site fidelity and do not undertake long migrations. In a series of mark-recapture studies in the Tamar estuary (England), Dando (2011) found that flounder mostly stayed within 200 m of the estuary and when experimentally displaced, showed homing behaviour. Similar results were found by Wirjoatmodjo and Pitcher (1984) who used acoustic tags to track flounder in the River Bann estuary in Northern Ireland; all flounder stayed within 400 metres of their tagging site. In addition, Le Pichon et al. (2014) reported that freshwater summer movements of acoustic tagged flounder were less than <870 m, suggesting that this is a sedentary life phase. However, there is also variation between populations and individuals; Summers (1979) used Petersen discs to tag flounder in the Ythan estuary and found that although most recaptures were close to the estuary (no distance provided), some individuals migrated up to 75 and 150 km from the estuary. Therefore, flounder do have the potential to undertake relatively extensive coastal migrations. Due to their limited dispersal capacity and homing behaviour, it may be inferred that flounder are likely to be most affected by developments within their “home” area.
Few studies on the overlap of flounder (or other flatfish) and offshore renewables exist. Barbut et al. (2020) studied six flatfish species and the overlap of their breeding grounds with offshore wind farm developments in the North Sea (which included six UK sites; five in England and one in Scotland). They used particle tracking and hydrodynamic models to demonstrate that there was very little overlap between the known breeding grounds of flounder and the two UK offshore wind farm sites used in the study.
3.1.8 European smelt (Osmerus eperlanus)
Across their range, European smelt (called sparling in Scotland) have migratory and non-migratory populations. Non-migratory, obligate freshwater populations of this species are only found in Scandinavia. In the UK, smelt is a coastal species that migrates from coastal waters into estuaries and rivers to breed (Maitland & Lyle, 1996). There is no information available to determine how far from the coast smelt may occur. Spawning usually takes place between late February and early April, when adults find fast-flowing freshwater. Smelt eggs are adhesive and attach to substrate and vegetation (Lyle & Maitland, 1997; Falconier, 2021). The spawning period is often short, rarely lasting more than a week (Hutchinson & Mills, 1987). UK adult smelt are short-lived, with an average life span of 3 years. While smelt usually reach sexual maturity at 2 years, maturation at a younger age has been reported (Hutchinson, 1983). Data from the most studied Scottish population in the River Cree that flows into the Solway Firth, suggests that most individuals caught during spawning migration were aged 1+ (72.3 %). Smelt have very low tolerance of poor water quality and are therefore prone to population crashes (Hutchinson & Mills, 1987; Falconier, 2021). Stomach content analysis of smelt caught in Ireland found that the marine mysid Praunus neglectus was by far the most prevalent prey item (56 - 90 % of diet) but there was also evidence of piscivorous feeding (whiting Merlangius merlangus, sprat Sprattus sprattus), including also cannibalism (Doherty & McCarthy, 2004). The presence of these marine species in the smelt diet suggests that smelt spend considerable time feeding in the marine environment, however how far these excursions extend is unknown.
In Scotland, smelt is currently only found in three locations; the rivers Cree, Tay and Forth but historically there are records for at least 15 populations. In England, smelt is found in the River Thames and River Trent (Falconier, 2021). Maitland and Campbell (1992) suggested that the UK populations are constrained to their estuaries, and this seems to be true in case of the Irish populations (Quigley et al., 2004). In a study by Elliott et al. (2023) that looked at fisheries bycatch along the UK and continental coast, most captures of smelt were done very near the coast, further suggesting that smelt may be unlikely to move far offshore (Figure 10). However no detailed studies on this have been done and therefore it is still unclear how far offshore smelt from UK populations migrate and thus whether they might be impacted by offshore wind developments.
Smelt is an understudied fish and very little published literature exists on most aspects of its biology and even less relating to movement ecology beyond descriptions of spawning migrations. One tracking study from England by Moore et al. (2016) was identified that was mainly focused on the freshwater movements of smelt, however it did record individuals moving out to sea. The fish showed a rapid movement out to the coastal zone and did not seem to spend long in the estuary zone.
3.1.9 Allis shad (Alosa alosa)
Allis shad are anadromous, spending most of their life in the marine environment and spawn in freshwater. Most allis shad sexually mature between three and eight years. Spawning takes place in late spring (April to June) and involves shoals of shad congregating at night. Clean gravel is the preferred spawning substrate but no nests are constructed, instead eggs are laid above the gravel. Most allis shad are semelparous and die after spawning, but some individuals return to the sea following spawning. Juveniles normally move to the estuaries and the sea towards the end of their first year or during their second year. Allis shad tend to be planktivorous (Maitland and Hatton-Ellis, 2003). It has been noted that there is only one confirmed spawning population of allis shad left in the UK, in River Tamar (England). However, there is some evidence that allis shad may spawn in or near the Solway Firth area, as spent adults (adults which have spawned) and allis/twaite shad hybrids have been recovered as by-catch in this area from stake nets set to capture Atlantic salmon (Etheridge, 2011).
The existing studies of the movement ecology of allis shad have focused on freshwater spawning migrations with very few studies examining their movement ecology and habitat use in marine waters. However, it is thought that they use coastal areas and are found in pelagic habitats (Maitland and Hatton-Ellis, 2003). A survey in France found that allis shad seem to have a preference for water depths of 10 - 20 m but have also been found at a depth of 150 m (Taverny 1991 in Maitland & Hatton-Ellis 2003). A review of 13 years of fisheries bycatch data by Elliott et al. (2023) included records of Allis shad; captures were most likely on the French coast and English Channel (Figure 10). No captures were recorded near the Scottish coast and only a few recorded near Shetland. However, it should be noted that the different gear types used in the data set are likely to significantly alter the catchability of specific species and therefore this data set may not be fully representative of the real distribution. Additionally, the presence of allis shad may have been missed by trawling studies due to their depth preference or the relatively low population size which would make the likelihood of catching shad unlikely.
3.1.10 Twaite shad (Alosa fallax)
The Twaite shad is closely related to the allis shad and the two species can hybridise. Despite being usually a marine species, there are some non-migratory freshwater populations in European lakes, including one in Ireland. Similar to the allis shad, twaite shad spend most of their life in the marine environment but return to freshwater to spawn. Mature adults usually congregate in estuaries in late spring before moving upstream to spawn in May and June. Males usually mature earlier than females at around 3 years, while females mature at around 5 years. Spawning usually takes place in the lower reaches of large rivers, above clean gravel substrate, however migrations further much upstream have been reported in some rivers. No nests are built, instead the eggs are laid above the substrate and subsequently sink down onto the substrate. The eggs hatch very quickly (4 - 6 days) and the juvenile fish move downstream to upper estuaries to start feeding. Twaite shad are iteroparous (can reproduce multiple times). Twaite shad can be both planktivorous and piscivorous (Maitland & Hatton-Ellis, 2003).
Twaite shad spawning populations are thought to only occur in southern England and Wales, most notably in the Severn estuary area (Aprahamian et al., 1998; Etheridge, 2011). However, spent adults and allis/twaite shad hybrids have been encountered in the Solway Firth area, which may suggest a spawning population in this region, although there is no clear evidence for this currently (Etheridge, 2011). Studies from mainland Europe suggest that there may be some level of natal river homing and population structuring in this species (Alexandrino et al., 2006).
Little is known about the twaite shad’s marine habitat use, however, it is thought that they are mainly found in pelagic coastal habitats (Maitland and Hatton-Ellis, 2003). The most extensive tracking study in UK waters was done by Davies et al. (2020) who tagged 73 upstream migrating adult twaite shad in the River Severn in the south-west of England. Of these, 58 were detected leaving the river and 12 were later detected approximately 200 km away. One tagged shad was detected in southern Ireland before returning back to the River Severn; a minimum migration distance of 950 km. This suggests that some twaite shad may move very long distances during their marine life migrations and use habitats far from the coast.
A survey in France suggested that twaite shad may have a preference for waters of 10 - 20 m water depth but fish were also found at depths of up to 110 m (Taverny 1991 in Maitland & Hatton-Ellis, 2003). Aprahamian et al. (2003) reports the species occurring at depths of 10 to 110 metres. A review of 13 years of fisheries bycatch data by Elliott et al. (2023) recorded twaite shad along the east coast of the UK, with more captures in English waters (Figure 10). Some captures were also recorded west of Scotland. However, it should be noted that the different gear types used in the data set are likely to significantly alter the catchability of specific species and therefore this data set may not be fully representative of the real distribution. Additionally, presence of Allis shad may have been missed by trawling studies due to their depth preference or the relatively low population size which would make the likelihood of catching shad unlikely.
3.1.11 Summary
Overall, there are very little data available on the marine space use and migration routes of UK diadromous fish. The most extensive data are available for Atlantic salmon but in comparison very little is known about the movements of the other species. Most of the data available are predominantly point capture or recapture data, which provide information on the presence, and in some cases the origin of the individual (mark-recapture studies), however it does not inform the actual or estimated migration routes undertaken. Depth use (which may influence potential offshore renewable impacts) of species during their marine life stages is available for some species, but only limited records exist for most. These data are summarised in Table 2.
Species |
Marine distribution (Y/N/P) |
Depth use (Y/N/P) |
Key references |
---|---|---|---|
Salmo salar |
P |
Y |
Gilbey et al., 2021; Holm et al., 2000; Newton et al., 2021; Mcilvenny et al., 2021; Green et al., 2022; Marine Directorate |
Salmo trutta |
P |
Y |
Kristensen et al., 2019a,b & c; Eldoy et al. 2017; Marine Directorate |
Anguilla anguilla |
P |
Y |
Righton et al., 2016; Wright et al., 2022; Elliott et al., 2023 |
Gasterosteus aculeatus |
P |
Y |
Hislop,1979; Marine Directorate |
Lampetra fluviatilis |
P |
N |
Elliott et al., 2021; Elliott et al., 2023 |
Petromyzon marinus |
P |
P |
Elliott et al., 2021; Elliott et al., 2023 |
Platichthys flesus |
P |
Y |
Elliott et al., 2023; Wirjoatmodjo & Pitcher, 1984; Summers, 1979 |
Osmerus eperlanus |
P |
N |
Elliott et al., 2023; Maitland & Campbell, 1992 |
Alosa alosa |
P |
P |
Elliott et al., 2023; Taverny, 1991 |
Alosa fallax |
P |
P |
Elliott et al., 2023; Taverny, 1991 |
3.2 Potential overlap of the migration routes of diadromous fish and Plan Option Areas
It is very likely that several of the POAs will have overlap with at least one species of diadromous fish; see Table 3. To make these overlap predictions, there are reasonable data available for salmon and anadromous brown trout, however data are very limited for the other diadromous fish species.
There is strong evidence that Atlantic salmon smolts and most likely adults as well, will transit through the POAs in Scottish waters. The most evidence exists for the east coast of Scotland, however there are some data available for north and west of Scotland. Additionally, considering the extent of marine space use it is feasible to assume that salmon are found in all POAs. While the overlap with most POAs has been shown, there are very little data available on the migration timings beyond coarse seasonal information. However, in relation to smolts, existing data suggests that smolts show a quick and directed movement towards their feeding grounds and therefore may be unlikely to spend long periods of time in the vicinity of POAs. Detailed tracking data does not exist for adult Atlantic salmon in Scottish waters, however, it may be likely that they spend more time in the coastal zone during the return migration while they search for their natal river, which could lead to extended exposure. Both smolt and adults are also very likely to be exposed to export cables in inshore waters as they move between freshwater and marine habitats.
There are less data available for the marine space use for sea trout in Scottish waters than Atlantic salmon, however the existing data suggests that potential overlap is likely, particularly for the sites that are closer to the coast. Additionally, due to the tendency of sea trout to remain in coastal waters for the duration of their marine migration, the temporal overlap and likelihood of exposure with export cable corridors associated with offshore wind farm sites are likely to be higher. There is a clear need for more data for sea trout, and acoustic tracking studies would be particularly informative.
European eel undertakes the longest migration of the 10 focal fish species in this review. Despite this and its threatened status, there are no marine tracking studies of eel from Scottish or UK waters. We know that eels migrate from and to the Sargasso Sea, however the exact routes taken are unclear for Scottish populations. Additionally, as it is not possible to track the juvenile glass eel stage, these data are not available. However, considering how widely eels are found in Scottish rivers, it is reasonable to assume that they are migrating widely in Scottish marine waters and therefore likely to encounter POAs and also export cables in the inshore waters. The temporal aspect of eel migration is not well known either and therefore it is difficult to estimate the extent of exposure to POAs and export cables. It is however likely to be higher for the larval stages that are weaker swimmers.
Although three-spined stickleback in the UK are often considered to be freshwater only, there are some data from Scottish waters to suggest that they are found, often in large numbers, quite far from land. Trawling studies have shown that three-spined stickleback are found in certain POAs so some overlap is confirmed. However, the existing sampling has been fairly limited spatially (mostly focused on the east coast) and temporally (only sampling during certain time of year), and it may be that if more sampling was undertaken, this species could be found in many more areas. Due to the very limited information on the marine use of European stickleback populations, while overlap is confirmed in some POAs, it is not possible to speculate how likely it may be in many of the other areas. As all existing data are from trawling studies, it is also not possible to say anything about the temporal aspect or the full extent of these marine migrations – therefore it is not currently known when stickleback move into the marine environment, or the duration and spatial extent of the migrations.
What we know of river and sea lamprey marine movements in Scottish and UK waters is limited to just a few bycatch studies. Additionally, very little information is available elsewhere globally which makes it very challenging to estimate the potential extent of overlap with offshore renewables. However, the bycatch data suggest that there is potential for overlap on some of the sites at least and lampreys have been caught >100 km from land, suggesting that they do have the capacity to migrate to an extent that would take them near offshore developments. River lamprey that remain in close proximity to the coast are unlikely to encounter POAs fairly close to the coast. This does however put them within the range of export cables if these overlap with rivers and estuaries where lamprey are found. Additionally, temporal overlap may be high if lamprey remain within the coastal waters.
European flounder has a ubiquitous distribution around the British Isles and therefore is very likely to overlap with POAs in all Scottish waters. They are more common closer to land so any POAs within ~50 km of land and subsea export cables are more likely to have overlap than developments that are further offshore. As flounder are more sedentary than the other focal species, it is also much more likely that the potential temporal overlap will be higher if they are in the vicinity.
The least amount of information available for fish movement in the marine environment is for European smelt, allis shad and twaite shad. Therefore, it is very difficult to estimate how likely and where any potential overlap would occur. These species are very rare however, so their limited freshwater distribution might provide some information on which POAs may be more likely sites of overlap. For example, in Scotland the two shad species are only found in the Solway Firth area on the southwest coast and therefore fish from these populations may be unlikely to overlap with POAs on the east coast although some evidence does exist that they are capable of long migrations. However, as we do not know enough about the marine migration of these species, it is not possible to speculate how extensive are their migrations. Some studies suggest that they may be commonly found in estuaries, potentially increasing their likelihood of overlap with export cables in estuaries where these species are found. Additionally, shad from other UK populations and possibly from continental Europe could migrate into Scottish waters as there is evidence from bycatch data that twaite shad especially has been caught in the east coast of Scotland. No detailed temporal data exists for the marine migrations and therefore it is not possible to say to what extent exposure might happen.
Table 3: The 10 study species and their likelihood (L) of overlap with the 28 Plan Option Areas based on evidence from literature and expert opinion. Assessment of confidence (C) in this likelihood (L) is also included; this is High if there is direct evidence for overlap, Medium if there is evidence for species presence in a nearby area and therefore it is reasonable to assume that overlap with the nearby development area may also occur, Low if there is only very limited marine data available. Apart from the High confidence columns, there is a high level of extrapolation required in assessments of confidence and likelihood, resulting from the limited evidence available, and therefore this table should be considered as expert opinion only. For the data-deficient species, it would be highly speculative to assess overlap so in these cases areas have been assigned as Unknown. Numbers in individual species columns refer to references listed below. H=High, M=Medium, L=Low, U=unknown, NA=not applicable.
Species |
Salmo salar |
Salmo trutta |
Anguilla anguilla |
Gasterosteus aculeatus |
||||
---|---|---|---|---|---|---|---|---|
L |
C |
L |
C |
L |
C |
L |
C |
|
POA |
||||||||
W1 |
H 1 |
H |
M |
M |
H1 |
M |
U |
NA |
NE4 |
H 2 |
H |
M |
M |
H 1 |
H |
U |
NA |
N3 |
H 2 |
H |
M |
M |
H 1 |
H |
U |
NA |
N2 |
H 2 |
H |
M |
M |
H 1 |
M |
U |
NA |
N1 |
H 1, 13 |
H |
M |
M |
H 1,12 |
M |
U |
NA |
NE2 |
H 3,6 |
H |
M |
M |
M 8 |
M |
M 4 |
M |
NE3 |
H 4,13 |
H |
M 4 |
M |
M |
L |
H 4 |
H |
NE4 |
H 4 |
H |
H 4 |
H |
M 1 |
M |
H 4 |
H |
NE8 |
H 4 |
H |
M |
L |
L |
L |
H 4,10 |
H |
NE6 |
H 4 |
H |
M |
L |
M |
M |
H 4 |
H |
NE7 |
H 4,6 |
H |
H 4 |
H |
L |
L |
H 4,10 |
H |
E2 |
H 4,6 |
H |
H 4 |
H |
L |
L |
H 10 |
H |
E3 |
H 3,4 |
H |
H 4 |
H |
M 9 |
H |
H 4 |
H |
E1 |
H 4 |
H |
H 4 |
H |
L |
L |
H 4 |
H |
NE1 |
H 6 |
H |
M |
M |
M 1 |
M |
H 10 |
H |
16 |
H 5,14,15 |
H |
H 7 |
M |
M |
M |
U |
NA |
17 |
H 4 |
H |
M 4 |
M |
M 1 |
M |
H 4 |
H |
18 |
H 4 |
H |
M 4 |
M |
M 1 |
M |
M 4 |
H |
19 |
H 1,4 |
H |
H 4 |
H |
M |
L |
H 4,10 |
H |
20 |
H 1,4 |
H |
M 4 |
M |
M |
L |
H 4,10 |
H |
21 |
H 4,6 |
H |
H 4 |
H |
L |
L |
H 4,10 |
H |
22 |
H 4,6 |
H |
H 4 |
H |
L |
L |
H 4,10 |
H |
23 |
H 4,6 |
H |
H 4 |
H |
L |
L |
H 4,10 |
H |
24 |
H 4,6 |
H |
H 4 |
H |
L |
L |
M 4,10 |
M |
25 |
H 4,6 |
H |
H 4 |
H |
L |
L |
M 4,10 |
M |
26 |
H 4,6 |
H |
H 4 |
M |
L |
L |
H 10 |
H |
27 |
H 6 |
H |
M |
M |
L |
L |
M 10 |
M |
28 |
H 6 |
H |
M |
M |
L |
L |
U |
NA |
Species |
Lampetra fluviatilis |
Petromyzon marinus |
Platichthys flesus |
|||
---|---|---|---|---|---|---|
L |
C |
L |
C |
L |
C |
|
POA |
||||||
W1 |
U |
NA |
U |
NA |
H |
M |
NE4 |
U |
NA |
U |
NA |
H |
M |
N3 |
U |
NA |
U |
NA |
H |
M |
N2 |
U |
NA |
U |
NA |
H |
M |
N1 |
U |
NA |
U |
NA |
H |
M |
NE2 |
U |
NA |
U |
NA |
H |
M |
NE3 |
U |
NA |
U |
NA |
H |
M |
NE4 |
U |
NA |
U |
NA |
H |
M |
NE8 |
U |
NA |
U |
NA |
M |
M |
NE6 |
U |
NA |
U |
NA |
M |
M |
NE7 |
U |
NA |
U |
NA |
M |
M |
E2 |
U |
NA |
U |
NA |
M |
M |
E3 |
U |
NA |
M 12 |
M |
M |
M |
E1 |
U |
NA |
L |
L |
M |
M |
NE1 |
H 11 |
H |
H 12 |
H |
H |
M |
16 |
U |
NA |
M 8 |
M |
H |
M |
17 |
U |
NA |
L |
L |
H |
M |
18 |
U |
NA |
L |
L |
H |
M |
19 |
U |
NA |
L |
L |
H |
M |
20 |
U |
NA |
L |
L |
H |
M |
21 |
L |
L |
L |
L |
M |
M |
22 |
L |
L |
L |
L |
M |
M |
23 |
L |
M |
L |
M |
L |
L |
24 |
L |
M |
L |
M |
L |
L |
25 |
L |
M |
L |
M |
L |
L |
26 |
L |
M |
L |
M |
L |
L |
27 |
L |
M |
L |
M |
L |
L |
28 |
L |
M |
L |
M |
L |
L |
Species |
Osmerus eperlanus |
Alosa alosa |
Alosa fallax |
||||
---|---|---|---|---|---|---|---|
L |
C |
L |
C |
L |
C |
||
POA |
|||||||
W1 |
U |
NA |
U |
NA |
U |
NA |
|
NE4 |
U |
NA |
M 12 |
L |
U |
NA |
|
N3 |
U |
NA |
H 12 |
H |
U |
NA |
|
N2 |
U |
NA |
M 12 |
L |
U |
NA |
|
N1 |
U |
NA |
U |
NA |
U |
NA |
|
NE2 |
U |
NA |
U |
NA |
H 12 |
H |
|
NE3 |
U |
NA |
U |
NA |
U |
NA |
|
NE4 |
U |
NA |
U |
NA |
U |
NA |
|
NE8 |
U |
NA |
H 12 |
H |
H 12 |
H |
|
NE6 |
U |
NA |
H 12 |
H |
H 12 |
H |
|
NE7 |
U |
NA |
M 12 |
H |
M 12 |
H |
|
E2 |
U |
NA |
U |
NA |
H 12 |
H |
|
E3 |
U |
NA |
U |
NA |
M 12 |
M |
|
E1 |
U |
NA |
U |
NA |
H 12 |
H |
|
NE1 |
L |
M |
M 12 |
H |
H 12 |
H |
|
16 |
U |
NA |
U |
NA |
U |
NA |
|
17 |
U |
NA |
H 12 |
H |
H 12 |
H |
|
18 |
U |
NA |
H 12 |
H |
H 12 |
H |
|
19 |
U |
NA |
U |
NA |
M 12 |
M |
|
20 |
U |
NA |
U |
NA |
M 12 |
M |
|
21 |
U |
NA |
U |
NA |
M 12 |
M |
|
22 |
U |
NA |
U |
NA |
M 12 |
M |
|
23 |
U |
NA |
U |
NA |
M 12 |
M |
|
24 |
U |
NA |
U |
NA |
H 12 |
H |
|
25 |
U |
NA |
U |
NA |
M 12 |
M |
|
26 |
U |
NA |
U |
NA |
U |
NA |
|
27 |
U |
NA |
U |
NA |
U |
NA |
|
28 |
U |
NA |
U |
NA |
U |
NA |
References for Table 3: 1: Malcolm, I. A., Godfrey, J., & Youngson, A. F. (2010). Review of migratory routes and behaviour of Atlantic salmon, sea trout and European eel in Scotland's coastal environment: implications for the development of marine renewables. Marine Scotland Science ; 2: Gilbey, J., Utne, K. R., Wennevik, V., Beck, A. C., Kausrud, K., Hindar, K., ... & Verspoor, E. (2021). The early marine distribution of Atlantic salmon in the North‐east Atlantic: A genetically informed stock‐specific synthesis. Fish and Fisheries, 22(6), 1274-1306; 3: Holm, M., Holst, J. C., & Hansen, L. P. (2000). Spatial and temporal distribution of post-smolts of Atlantic salmon (Salmo salar L.) in the Norwegian Sea and adjacent areas. ICES Journal of Marine Science, 57(4), 955-964; 4: Marine Directorate (2023). ScotMER conference - Diadromous fish session; 5: Lilly, J. M. (2023). The behaviour of Atlantic salmon (Salmo salar) on first migration to sea (Doctoral dissertation, University of Glasgow); 6: The expert panel workshop.; 7: Diego del Villar, pers. comm.; 8: https://sac.jncc.gov.uk/species/ (showing that there are populations in nearby rivers, therefore it is quite likely that they will overlap with close developments); 9: Barry, J., Bodles, K. J., Boylan, P., & Adams, C. E. (2015). Historical change in the European eel population in the Foyle estuary, Northern Ireland. In Biology and Environment: Proceedings of the Royal Irish Academy (Vol. 115, No. 2, pp. 137-142); 10: Hislop, J. R. G. (1979). Preliminary observations on the near‐surface fish fauna of the northern North Sea in late autumn. Journal of Fish Biology, 15(6), 697-704; 11: Elliott, S. A., Deleys, N., Rivot, E., Acou, A., Réveillac, E., & Beaulaton, L. (2021). Shedding light on the river and sea lamprey in western European marine waters. Endangered Species Research, 44, 409-419; 12: Elliott, S. A., Acou, A., Beaulaton, L., Guitton, J., Réveillac, E., & Rivot, E. (2023). Modelling the distribution of rare and data-poor diadromous fish at sea for protected area management. Progress in Oceanography, 210, 102924; 13: Godfrey et al. (2015). Depth use and migratory behaviour of homing Atlantic salmon (Salmo salar) in Scottish coastal waters. ICES Journal of Marine Science, 72(2), 568-575.; 14: Green et al. (2022). Evidence of long-distance coastal sea migration of Atlantic salmon, Salmo salar, smolts from northwest England (River Derwent). Animal Biotelemetry, 10(1), 3.; 15: Barry et al. (2020). Atlantic salmon smolts in the Irish Sea: first evidence of a northerly migration trajectory. Fisheries Management and Ecology, 27(5), 517-522.
3.2.1 Diadromous fish marine space use - key evidence gaps
One of the clearest evidence gaps highlighted by the literature review and expert panel discussions was the lack of information available on the marine habitat use and distribution of all 10 focal fish species, and consequently any confidence in assessment of overlap with the POAs. Most of the information available is focused on Atlantic salmon, but even for this species, the available data are limited to certain locations and life stages. Understanding the likelihood of connectivity and potential impact pathways between the 10 diadromous species and POAs is of critical importance as it underpins both the assessment process as well as guiding research. Therefore, this should be considered the highest priority. Gaining knowledge on the distribution of these species and of potential impact pathways with offshore renewables should form the first step in any research programme for any individual species.
This topic can be addressed using a combination of several methodologies to build a comprehensive picture of the marine distribution of diadromous fish. For this evidence gap, four potential methods suitable to help answer this question are presented. For each one, the method is briefly introduced and suggested study approaches are presented, including discussion on spatial and temporal scales of data collection, feasibility and challenges.
3.2.2 eDNA
Environmental DNA (eDNA) has the potential to provide quick and relatively affordable information on species’ presence. eDNA methods are particularly accurate in freshwater habitats but have also been very successfully used in marine environments for species identification, e.g. searching for target species or for community assessments through metabarcoding (i.e. Gold et al., 2022). Additionally, it has been shown that eDNA degrades quickly (days) in sea water and therefore positive detection is a sign of recent presence of the species (Thomsen et al., 2012). For this present study, we recommend a metabarcoding approach as the aim is to target the 10 focal species. It is recommended that 4-5 litres of seawater are collected to ensure sufficient DNA capture in the sample (Valsecchi et al., 2021).
Spatial and temporal scales of data collection: Spatial coverage of this work would be most effective if sampling were extensive, covering a number of key POAs. Sampling intensity will depend on the aims of the study programme and detail of information required. There are currently no clear recommendations for the sampling intensity in marine environments (Gold et al., 2022). Goldberg et al. (2016) recommend conducting a pilot study for each new wind farm application to account for variation in detection probability due to concentration of eDNA in the sample, capture efficacy, extraction efficacy, sample interference and assay sensitivity. They also recommend collecting multiple samples per sampling site to account for false negatives and estimation of detection probabilities. Repeated sampling through the year is recommended to account for temporal changes, and especially during the key migration times of the study species to ensure that sampling takes place when target species are most likely to be present.
Feasibility and challenges: The main limitation of eDNA is that it is still mainly used for qualitative analysis, although in certain habitats it has been used to gather quantitative information as well. Collection of the water sample itself and processing of eDNA samples for laboratory analysis is simple, however the challenge in marine sampling is accessing sample sites which may be several hundred kilometres from the shore. It is our recommendation that in addition to targeted sampling, where possible sample collection is combined with other survey work or with commercial fisheries. eDNA water samples could be collected by commercial fisheries operators to increase the number of sampling points around Scotland; there are examples of simple tools to collect eDNA on board of trawl vessels (for example see Maiello et al., 2022 where the authors sampled the water in the holding tank on a fishing boat). eDNA samples could be collected two ways; from the ship holding tanks as mentioned before, which would give an accurate representation of the catch, or directly from the sea which would allow for a more representative sample of the community composition. The availability of primers for the lab analysis for all species should also be ensured and thus if these are not available, development of these should be in the budget.
3.2.3 Telemetry
Telemetry methods have the potential to provide very valuable fine-scale information about important migration routes and timings. However, it is an expensive technique and depends on extensive and carefully targeted receiver coverage, in addition to previous expertise of the technology. Due to the high cost and logistical challenges, it is recommended that collaborative projects are undertaken whenever possible, to maximise the amount of receiver coverage.
Species included: Atlantic salmon, brown trout (satellite & acoustic tags), twaite shad, allis shad, sea lamprey, flounder, eel, sparling (acoustic tag)
Two possible options for telemetry studies are satellite tagging of salmon or sea trout adults (most likely kelts) or very carefully planned acoustic telemetry study with salmon or sea trout smolts/adults. For comprehensive reviews on the use of telemetry for tracking fish see Matley et al., 2022 and Thorstad et al., 2013.
Satellite tagging: The main benefit of satellite telemetry is that it does not require extensive receiver coverage. However, the major limitation of satellite telemetry is the size of the tag and thus only larger fish can be included in these types of study. Adult salmon and some sea trout would be large enough to carry satellite tags and considering their importance, they would make very good focal species for this study. The fish would be easiest to catch as post-spawning (kelts), either in nets or Wolff traps in fresh water where this facility is available. There have been international studies on kelt movements showing detailed pathways of the migration routes (i.e. Rikardsen et al., 2021), but there has been no published study that included Scottish fish - although Godfrey et al. (2015) satellite tagged adult salmon in northern Scotland. We suggest a large-scale Atlantic salmon and sea trout kelt satellite tagging project that would include at least 10 rivers (4 on the west coast, 4 on the east coast and 2 from the north coast) and with a minimum of 15 tags per river. This would provide a relatively wide geographical coverage and relatively robust sample size, while still taking into consideration the high cost of satellite tags.
Acoustic tagging of smolts/adults: In the last 4 years there have been several large-scale salmon smolt tracking projects (using acoustic telemetry) around Scotland, however the work is still largely unpublished (due to the field work or data analysis currently planned or ongoing). These projects have revealed, for the first time, the overall directionality of the northern migration of salmon smolts, however these data still lack a lot of detail. Future studies should expand on these studies and aim to fill any remaining evidence gaps. Additionally, most of the large-scale tracking has been undertaken on the west coast of Scotland so future work would be most effective by targeting the areas that have received less attention so far. An acoustic telemetry project that has receivers placed around the edges of or within an offshore development would provide clear evidence of an overlap through the detection of individuals of the focal species. This sort of a study could have two approaches; either targeting one or two rivers which may lead to a low chance of overlap, or, attempting to tag in multiple rivers which may give a more realistic picture of the likelihood of overlap and origin populations of overlap in that region. The best study areas would likely be a development area near the coast and targeting rivers that are near that development. Considering the high loss rates of salmon smolts in freshwater, tagging should be done close to the estuary to minimise the known high freshwater mortality (Thorstad et al., 2012; Lothian et al., 2018) and appropriate sample size used to account for this. A minimum of 80 fish per river should be tagged. In the first instance, selecting rivers that are a Special Area of Conservation (SACs) for salmon is recommended. We suggest the River Spey, River Dee and River South Esk. Salmon is the primary reason for the SAC designation status in these rivers and they are located near many of the POAs, therefore fish from these rivers are potentially likely to overlap with the developments. The exact receiver array design will be dependent on available budget, however as a general approach a ‘leaky line’ with receivers spaced approximately 1 km apart is recommended. We suggest multiple long lines ~30 km length, particularly targeting POAs NE2-NE8 and 17-18 (Figure 1). While there has been previous work in this area and overlap of POAs and many diadromous fish species has been shown already, very little detailed information on the migration timings exists. As this is an area that has the most POAs present, it is a key area where diadromous fish may come into contact with multiple developments, and therefore more detailed information on the migration routes is required. Another potential approach would be focusing on POAs E1-E3 and 21-28, as these are areas where much less information is available.
Spatial and temporal scales of data collection: Ideally, both of the above telemetry studies (acoustic and satellite tagging) would run for a minimum of two years to account for temporal variability but even one year of data would be very valuable owing to the lack of such information. As mentioned above, these studies should have wide spatial coverage including rivers from the east, west and north of Scotland. However initially, it is recommended that work focuses on the east coast of Scotland as this is the area that currently has most planned offshore developments. Additionally, it is likely that at least Atlantic salmon from England, Wales and possibly Northern Ireland may overlap with POAs within Scottish waters during their migration north and therefore there is an opportunity to collaborate beyond Scottish rivers.
Feasibility and challenges: Acoustic telemetry is very useful for examining specific sites whereby receivers are placed in areas proposed for development. It is one of the best methods currently to track animal movements and a carefully planned study design could provide insights into the animals’ behaviour. However, due to the relatively low detection ranges (200 – 1500 metres depending on tag type and environmental conditions; see Kessel et al., 2014 and Reubens et al., 2019), the potential detection area is limited and thus, it is likely tags may not be detected in the study area. In addition, careful consideration is needed when designing acoustic telemetry studies as the deployment and retrieval of acoustic receivers can be challenging, especially in deeper waters, further away from the coast and in areas with difficult hydrological conditions. Receiver deployment and recovery relies on using boats and therefore it can be costly, depending on the quantity and location of receivers. In addition, it is possible that equipment and data could be lost during the study. For example, as acoustic telemetry receivers are moored on the seabed, they are vulnerable to being caught by commercial fishing bottom trawls. Therefore, careful consideration and contingency planning needs to be undertaken when evaluating feasibility of a study, to minimise the risk of receiver snagging and loss. This must include, for example, good communication with local stakeholders and study designs that can endure loss of some receivers.
Another consideration of all telemetry methods is that placing a tag on an animal (externally or internally) is an inherently stressful process. Even with carefully controlled Standard Operating Procedures (SOP)s and complying with animal welfare laws, guidelines and policies (e.g. obtaining a UK Home Office licence), there is a possibility that tagging could have an effect on the tagged animal’s behaviour – it should be considered that while the assumption is that the behaviour of the tagged animal is representative of the rest of the population, this may not be valid. With regards to the kelt study specifically, capturing kelts can be very difficult and time consuming so reaching the target sample size may be challenging. Additionally, some kelts will have a poor body condition (due to spawning) and therefore may not be suitable for tagging. Potential negative impacts of tagging and other considerations are discussed in Thorstad et al., 2001, Caputo et al., 2009, and Klinard and Matley 2020. However, while some negative impacts of tagging may exist, it remains one of the best ways to study movement ecology of aquatic species. Additionally, there is evidence to show that the potential negative effects of tagging are minimal and short-term (Klinard et al., 2018; McCabe et al., 2019).
3.2.4 Trawling surveys (scientific)
Marine Directorate scientific smolt trawling surveys have provided valuable data on the marine distribution of Atlantic salmon, sea trout and three-spined stickleback, providing proof of concept for this method. Despite the surveys targeting juvenile salmon, they have also provided novel information on the spatial distribution of sea trout and stickleback in marine waters. We recommend that these surveys be continued for 3-5 years to build a long-term dataset, with a suitable net type and depth that maximises the likelihood of capture of the other diadromous fish species, where feasible.
Spatial and temporal scales of data collection: It is recommended that the current survey efforts that have focused on the wider Scottish east coast and Moray Firth area should be expanded and where possible, targeted on the locations of PDAs. The current surveys have taken place during the smolt run in the spring. While it would be useful to collect data during other times of the year to build a more comprehensive dataset, we believe that considering the expense of these surveys, continuing to focus on the smolt run (April – June) when the likelihood of getting data on salmon smolts is the highest, is the best approach. Another time period worth considering is during the autumn (September – October) when there may be autumn migrating smolts, for which there is currently little information available.
Feasibility and challenges: The spot sampling approach of this recommended method will provide a good overview of the fish species distribution during the months of April – June. However, unless it is financially feasible to conduct trawling studies throughout the year, the changing distribution of species throughout the year will not be captured. Additionally, this approach will provide point data at specific locations, and will therefore miss the nuance of species movement. However, it would still provide valuable data on the presence/absence of species of interest. Funding will always be a challenge for any work requiring boat time.
3.2.5 Data from commercial fisheries
Commercial fisheries records could provide further data on the distribution of the 10 focal fish species. Elliott et al. (2021 & 2023) highlighted the successful use of fisheries bycatch data to provide additional information on the spatial distribution of diadromous fish species in marine waters.
Spatial and temporal scales of data collection: Data received via this methodology would likely be opportunistic, so designing a clear study methodology would not be possible, and the limitations (such as different catching methods) would need to be considered when comparing results. Accurate location data in addition to catch records and equipment detail is required.
Feasibility and challenges: This study proposal relies on the cooperation from commercial fisheries. It is possible that volunteer interest will be low, even with a reasonable financial incentive. However, these opportunistic data points on species distribution will provide valuable data that would otherwise be very difficult and expensive to collect, making it a worthwhile effort to improve the evidence base.
3.3 Potential direct and indirect effects on Special Areas of Conservation (SAC)
Section 2.4 highlighted the challenges of identifying connectivity and potential impact pathways between qualifying features of Special Areas of Conservation (SAC) and offshore wind development.
In the context of this review, this extends to Atlantic salmon, sea lamprey and river lamprey, which are recognised as features of SACs. Allis shad and Thwaite shad are recognised features, but currently do not contribute to any SAC designation in Scotland. Freshwater pearl mussels (Margaritifera margaritifera) are also features of SACs in Scotland. Freshwater pearl mussels use salmon and trout as hosts during their early development (glochidia, juvenile stages of mussels attached to the gills of host Salmo species) and may potentially be indirectly impacted by offshore development through changes to the host population. In Scotland, Atlantic salmon and trout are both hosts for freshwater pearl mussels (Clements et al., 2018), this makes the Scottish populations distinct compared with other geopolitical regions, where mussels appear to use one or other of the Salmo host species.
There are 17 SAC sites across Scotland for which Atlantic salmon are a feature, six SAC sites for sea lamprey, six SAC sites for river lamprey, and19 SAC sites for freshwater pearl mussel (summarised in Table 4).
Site condition monitoring (SCM) for SACs is undertaken by NatureScot (Tweed Estuary SAC monitoring undertaken by Joint Nature Conservation Committee (JNCC)). The SCM determines the condition of the qualifying features within SACs, whether the feature is likely to maintain itself in the medium to longer term under the current conditions. Monitoring the status of the populations of species’ detailed above forms part of the reporting requirements and includes an assessment of the populations and the factors that may negatively or positively affect features. These pressures can reveal why a feature is in an unfavourable condition, for example due to impacts to water quality and habitats
The classifications for SACs based on SCM are:
Favourable maintained – An interest feature should be recorded as maintained when the conservation objectives were being met at the previous assessment, and are still being met.
Favourable recovered – A feature of interest can be recorded as having recovered if it has regained favourable condition, having been recorded as unfavourable at the previous assessment.
Favourable declining – The attribute targets set for the natural feature have been met, but evidence suggests that its condition will worsen unless remedial action is taken.
Unfavourable recovering – A feature of interest can be recorded as recovering after damage if it has begun to show, or is continuing to show, a trend towards favourable condition.
Unfavourable no change – An interest feature may be retained in a more-or-less steady state by repeated or continuing damage – it is unfavourable but neither declining or recovering. In rare cases, an interest feature may be unable to regain its original condition following a damaging activity, but a new stable state might be achieved.
Unfavourable declining – Decline is another possible consequence of a damaging activity. In this case, recovery is possible and may occur either spontaneously or if suitable management input is made.
Partially destroyed – It is possible to destroy sections or areas of certain features or to destroy parts of sites with no hope of reinstatement because part of the feature itself, or the habitat or processes essential to support it, has been removed or irretrievably altered. In these cases, the remainder of the feature is given an assessed condition.
Totally destroyed – The recording of a feature as destroyed will indicate the entire interest feature has been affected to such an extent that there is no hope of recovery, perhaps because its supporting habitat or processes have been removed or irretrievably altered.
SAC Name |
Reported Feature Condition |
|||
---|---|---|---|---|
Atlantic Salmon |
River Lamprey |
Sea Lamprey |
Freshwater Pearl Mussel |
|
Berriedale and Langwell Waters (14) |
Favourable Maintained |
|||
Langavat (73) |
Unfavourable Recovering |
|||
Little Gruinard River (51) |
Favourable Recovered |
|||
River Bladnoch (16) |
Unfavourable Recovering |
|||
River Dee (28) |
Favourable Maintained |
Unfavourable No change (2003) |
||
River Naver (81) |
Favourable Recovered |
Unfavourable No change (2003) |
||
River South Esk (37) |
Unfavourable Recovering |
Unfavourable No change (2009) |
||
River Spey (94) |
Unfavourable Recovering |
Favourable Maintained (2011) |
Unfavourable Declining (2019) |
|
River Tay (98) |
Favourable Maintained |
Favourable Maintained (2007) |
Favourable Maintained (2011) |
|
River Thurso (99) |
Unfavourable Recovering |
|||
River Tweed (101) |
Favourable Maintained |
Favourable Maintained (2018) |
Unfavourable Declining (2018) |
|
Endrick Water (22) |
Unfavourable Recovering |
Favourable Maintained (2010) |
||
North Harris (39) |
Favourable Maintained |
Unfavourable No change (2014) |
||
River Borgie (81) |
Favourable Recovered |
Unfavourable No change (2014) |
SAC Name |
Reported Feature Condition |
|||
---|---|---|---|---|
Atlantic Salmon |
River Lamprey |
Sea Lamprey |
Freshwater Pearl Mussel |
|
River Moriston (83) |
Unfavourable No change |
Unfavourable No change (2018) |
||
River Oykel (66) |
Favourable Recovered |
Unfavourable No change (2015) |
||
River Teith (44) |
Unfavourable Recovering |
Favourable Maintained (2011) |
Unfavourable Declining (2011) |
|
Solway Firth (NA) |
Condition Not Assessed |
Condition Not Assessed |
||
Tweed Estuary (NA)* |
Present (Data Deficient) |
Present (Data Deficient) |
||
Abhainn Clais an Eas and Allt a' Mhuilinn (58) |
Unfavourable Declining (2014) |
|||
Ardnamurchan Burns (91 & 97) |
Unfavourable Declining (2014) |
|||
Mingarry Burn (10) |
Unfavourable Recovering (2014) |
|||
River Evelix (66) |
Unfavourable Declining (2014) |
|||
River Kerry (11) |
Favourable Maintained (2002) |
|||
River Moidart (77) |
Unfavourable No change (2014) |
|||
Ardvar and Loch a' Mhuilinn Woodlands (68) |
Unfavourable Declining (2014) |
|||
Foinaven (56) |
Unfavourable Recovering (2014) |
|||
Glen Beasdale (62) |
Unfavourable No change (2014) |
|||
Inverpolly (64) |
Unfavourable Declining (2016) |
|||
Rannoch Moor (26) |
Unfavourable No change (2010) |
For Atlantic salmon, the last reported SCM was undertaken in 2011 (Rivers and Fisheries Trusts of Scotland, 2014, at the time of writing, a recent SCM for Atlantic salmon had been undertaken, but the results were not available). This process assessed population status based on three pieces of information; (1) juvenile status, based upon electrofishing surveys undertaken by fisheries trusts; (2) rod catch data and; (3) surveys of fisheries managers. Recently, the assessment of juvenile populations has been harmonised across Scotland under the National Electrofishing Programme for Scotland (NEPS) (Scottish Government, 2020b). Further developments since the last SCM for Atlantic salmon include the development of a Pressures Tool (Fisheries Management Scotland, nd) which is currently being validated. In addition to assessing the status of salmon populations in SACs, Marine Directorate also undertakes an annual assessment of the status of the salmon populations in 173 catchment areas under the conservation of Salmon (Scotland) Regulations 2016. The status of these salmon stocks are compared against agreed international benchmarks with the aim of maintaining stocks at sustainable levels and are grouped into categories 1, 2 and 3. The recent classification of the 2024 angling season highlighted that the majority of stocks (112 out of 173) are thought to be in poor conservation status and these are spread throughout the country.
SCM for lamprey is undertaken following the protocols in JNCC (2015). SCM is undertaken at the site level, and thus no reporting year and associated report exists for Scotland (like that for Atlantic salmon), however, summaries of the Scottish SACs are provided in Article 17 reports (sea lamprey, JNCC (2019a); river lamprey, JNCC (2019b)). The most up-to-date SAC condition for sea and river lamprey are provided in Table 4.
For freshwater pearl mussels, surveys follow JNCC protocols (JNCC, 2015), which involve an assessment of the size of the mussels present at the site. Estimates of juvenile mussels being recruited back to the population are made and an assessment of status of the population is made.
3.3.1 Data Sources and Handling
As with all monitoring of a population, information gathered provides a snapshot of the population status at the time of the survey. Information about long-term trends can provide a greater insight into population status, but these data must be treated with caution, as there are multiple factors which can impact their accurate interpretation including, but not limited to; the effort employed to generate the data, the reasons underpinning the data collection (which can change over the course of the dataset), changes to methodology, and how data are recorded. Sources of information, which may form part of an assessment for potential impacts (direct and indirect) on features (specific to this review) which form part of an SAC designation have been summarised below (Table 5) . In addition, considerations of how these data are utilised have also been provided. Consideration of temporal correlation should also be considered. Care should be taken when interpreting how the data were collected, for example, in the Marine Directorate fishery catch dataset, a zero in the dataset could mean either there were no fish captured (a true zero) or that there was no catch return provided (a false zero). In the last few years, Marine Directorate has started to record the amount of effort (in the form of angler days) in the fishery catch dataset, but this is only available since 2019. Finally, some fishery Districts do not align well with SAC extent, for example, the Endrick Water SAC falls within the Clyde District.
3.3.1.1 Atlantic salmon and sea trout
Marine Directorate has been collecting the number of Atlantic salmon and sea trout captured by angling since 1952. These data form a significant and useful source to assess long term changes in the population of these species at a moderately fine spatial scale. Fish caught are reported at the “District” level, which is akin to a catchment in some areas (e.g. on the east and south west coast) but can be a combination of smaller catchments (e.g. on the north west coast and the islands). SACs where Atlantic salmon and/or freshwater pearl mussel are a qualifying feature have been linked with the reporting District and the long-term data have been displayed (Appendix 1).
Atlantic salmon may mature in one or more than one year (2 or 3 years) in the marine environment, respectively referred to as single sea winter (1SW or grilse) and multi-sea winter (MSW) fish. The multi-sea-winter component of the Atlantic salmon population is a UK Biodiversity Action Plan priority fish species. It is therefore important to look at the different components of the salmon stock in terms of number of sea winters (1SW vs MSW) and the timing of the fish being captured (Spring is January to June, Summer is July to August and, Autumn is September to December) in the freshwater environment (these data have been summarised in Appendix 1).
Information about juvenile populations of Atlantic salmon and trout are undertaken annually by Fisheries Trusts across Scotland. To provide an accurate reflection of annual change in the state of juvenile Atlantic salmon, the NEPS was established in 2019. This provided a scientifically and statistically robust national programme to monitor trends in juvenile salmon abundance. These data are summarised annually in the NEPS reports to Scottish Government. Changes linked with the SACs have been summarised in Table 5.
3.3.1.2 River and Sea Lamprey
At present, there is no national data archive relating to trends in lamprey populations.
SAC Name |
Salmon Parr Trend |
Salmon Fry Trend |
---|---|---|
Berriedale and Langwell Waters |
||
Langavat |
||
Little Gruinard River |
||
River Bladnoch |
– |
– |
River Dee |
– |
↓ |
River Naver |
↓ |
↓ |
River South Esk |
– |
– |
River Spey |
↓ |
– |
River Tay |
– |
↓ |
River Thurso |
↓ |
– |
River Tweed |
– |
– |
Endrick Water |
↓ |
– |
North Harris |
||
River Borgie |
||
River Moriston |
– |
↑ |
River Oykel |
– |
– |
River Teith |
↑ |
– |
3.4 Potential impacts on diadromous fish populations of specific aspects of the development of offshore renewable energy production
A set of specific potential impacts on fish populations from the development of offshore wind energy production were identified through the literature. This is not a fully exhaustive list but instead the focus was on the key potential impacts. Further investigation of these potential impacts was undertaken through expert engagement workshops.
The potential impacts from the development of offshore renewable wind production were grouped and identified as:
- Sound and vibration
- Changes to light patterns
- Electromagnetic fields
- Novel habitat construction (physical barriers, sediment disturbance, predator-prey interactions and disease)
For each potential impact, a review of the literature is provided which includes a broad background and examples from the literature. Information and assessment of the available research by the expert panel is then provided, which is followed by species-specific accounts of studies focusing on the 10 focal fish species. An assessment by the authors of this report is provided on the likelihood and magnitude of the potential impact, considering the existing knowledge of species biology and ecology. Finally, emerging evidence gaps are highlighted and recommendations for future research are presented.
3.4.1 Sound and vibration
3.4.1.1 Fish hearing
In this report, sound and vibration refers to all forms of vibration emanating from any process across the entire life span of the project from surveying ground conditions (e.g. geotechnical surveys sediment testing during surveys) through the construction phase (e.g. pile driving) to operational phase (e.g. vibration through structures from blade rotation) and finally decommissioning. In aquatic environments, vibration is transmitted and interpreted by animals through two mechanisms, (i) sound pressure and (ii) particle motion. While some fish do respond to sound pressure, many aquatic organisms respond only to particle motion (like salmonids) (Popper & Fay, 2011; Ladich & Fay, 2013), see Figure 12.
Fish have specialised sensory organs for hearing including the inner ear and the lateral line system. It is likely that all fish can detect vibration (Ladich & Fay, 2013; Popper & Fay, 2019). Fish detect vibration primarily using particle motion and some fish also use sound pressure (Popper & Fay, 2019). Traditionally, fish have been divided into “hearing specialists” and “hearing generalists”, however instead of the two groups, it is more accurate to view fish sound detection as a continuum (Figure 12). Most fish can detect sound between 50 Hz and 500 Hz and those that can use sound pressure in addition to particle motion, can hear up to 1000 Hz or for some species even up to 4000 Hz (Popper & Fay, 2019). The presence of a swim bladder and how close it is to the inner ear, also plays a role in sound sensitivity. The 10 focal fish species in this review represent a range of physical adaptation from species with no swim bladder (river lamprey), to salmonids that do have a swim bladder but it’s far from the ear to the two shad species that have a connection between swim bladder and the ear, see Table 6. Due to this, Clupeids, which include the subfamily Alosinae that Allis shad and Twaite shad belong to, seem to have a much greater auditory range than many other fish species and can detect ultrasound (>20 kHz).
Lamprey (Order Agnatha – jawless fish) have received much less attention on their detection ability than teleost fish, but there are differences in the anatomy. Teleosts have three sensory maculae whereas lampreys have a macula communis which may be an evolutionary precursor to sensory maculae (Ladich & Popper, 2004; Mickle et al., 2019). The lamprey inner ear also has ciliary chambers that seem to cause fluid flow, this is a feature that is not found in other vertebrates (Popper & Hoxter, 1987). Maklad et al. (2014) found that sea lamprey ears have auditory hair cells and a large statolith located in a macula communis. The simpler structure of the lamprey inner ear may make them less sensitive to motion and ability to orient source of sound.
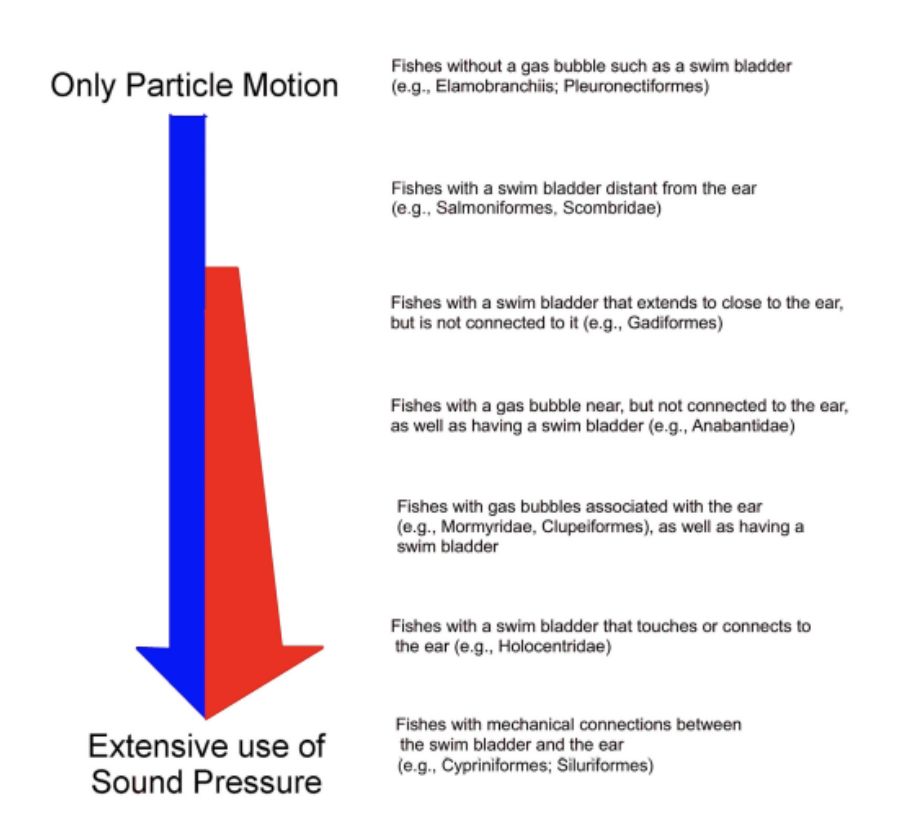
The effects of vibration and sound on fish can be divided into three categories: i.) primary effects which are severe, often fatal injuries (barotrauma), ii.) secondary effects which may have long-term implications for survival (i.e. deafness), and iii.) tertiary effects which are behavioural changes, such as avoidance of an area (Nedwell et al., 2003). While physical injuries are the most severe, impacts resulting in behavioural changes may also be significant, as it may lead to movement of fish away from migration routes, affect reproductive behaviour and interfere with communication (Popper & Fay, 2019). There are 30,000 extant fish species and at least 800 species are known to produce sounds which are used for a variety of purposes from mating to fighting, thus, any disruption by anthropogenic noise could have significant adverse effects (Bass & Ladich, 2008).
Species |
Auditory range |
Detection mechanism |
References |
---|---|---|---|
Atlantic salmon |
30 - 800 Hz |
Particle motion (swim bladder but far from ear) |
Hawkins & Johnstone, 1978; Harding et al., 2016 |
Brown trout |
20 - 1000 Hz |
Particle motion (swim bladder but far from ear) |
Nedwell et al., 2006 |
European eel |
11 - 400 Hz |
Particle motion (swim bladder but far from ear) |
Jerkø et al., 1989; Sand et al., 2000 |
Three-spined stickleback |
25 – 1000 Hz |
Particle motion (swim bladder but far from ear) |
Purser & Radford, 2011; Andersson et al., 2007 |
River lamprey |
Not known |
Particle motion (no swim bladder) |
- |
Sea lamprey |
50 - 300 Hz |
Particle motion |
Mickle et al., 2019 |
European flounder |
Not tested for flounder; dab and plaice detection range overlaps from ~20-300 Hz |
Particle motion (no swim bladder) |
Chapman & Sand, 1974 |
European smelt |
Not known |
Particle motion (swim bladder but far from ear) |
- |
Allis shad |
Likely similar to twaite shad (>60 kHz) |
Connection between ear and swim bladder |
- |
Twaite shad |
lower range unknown - >60 kHz |
Connection between ear and swim bladder |
Teague & Clough, 2014 |
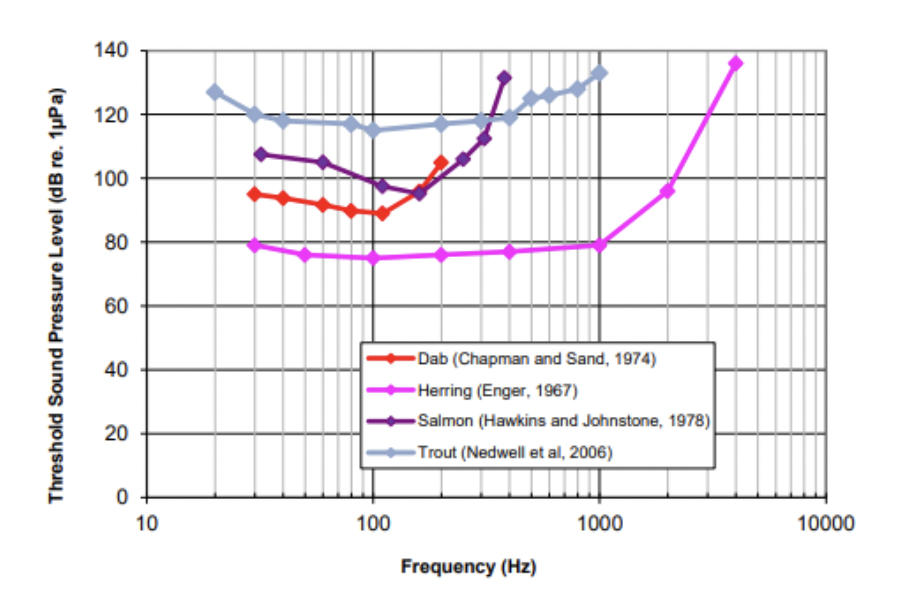
3.4.1.2 Particle motion
Particle motion is the movement (vibration) of small particles within the water and the mechanisms for detection by aquatic animals is moderately well understood. In simple terms detection of particle motion involves the movement of a hair or hair-bundle in the ear of the fish which triggers nerve impulses (Popper & Hawkins, 2018). Particle motion can be expressed as displacement (m), velocity (ms-1) or acceleration (ms-2). However, relatively little research in impact/effect studies has focused on the role of particle motion, with the focus mainly being on sound pressure. This is concerning, considering that most fish use particle motion as a primary method for sound detection. Sound pressure can be used as a proxy for particle motion, however this relationship is only valid under certain conditions which does not usually include shallow waters where offshore developments often take place (Nedelec et al., 2016). Nedelec et al. (2016) recommend that particle motion measurement should be considered at “depths less than 100 m and frequencies less than 1 kHz, and at distances from the source less than the Fraunhofer distance (distance where the near field transitions to the far field) or one wavelength, whichever is greater.” Therefore, to fully measure the potential impact of noise from aquatic anthropogenic sound, particle motion sensors should be used. The likely reason for the lack of particle motion studies in the field is the complexity and cost of the required equipment, which has only recently become commercially available.
There are some examples of field measurements of particle motion, using purpose-built, bespoke particle motion sensors. One such study was conducted by Sigray et al. (2022), where the sensor had a submerged near-neutral buoyancy sphere with an accelerometer; with the sphere moving with the water particles and giving a measurement of the particle motion. In their study, Sigray et al. (2022) measured the particle motion produced from pile driving under two different mitigation methods (air isolated steel barrier with an internal bubble screen and a stand-alone bubble screen) and they found that there was a significant reduction gained through using these methods. Using a combination of mitigation methods, a broadband level (re 1 μm/s2) reduction of 26 dB was achieved. When looking at the spectral analysis, they found that using bubble curtains was particularly efficient in reducing noise in the 30 to 1000 Hz range.
3.4.1.3 Potential impacts from offshore developments
When assessing the potential impacts from offshore wind developments, it is useful to consider both the ambient soundscape and the anthropogenic noise. Aquatic environments have a level of ambient vibration, created by environmental (wind, waves) and biotic (organisms) sounds. The ambient vibration will, therefore, vary depending on habitat, time of day and weather conditions. Anthropogenic noise may be an issue if it is louder than the ambient background noise. As an example, Mueller-Blenkle et al. (2010) recorded ambient noise for their study system in a bay in western Scotland and found that ambient noise levels varied from 110 dB re 1 uPa on a calm day to 119 dB re 1 uPa during moderate to strong winds.
3.4.1.4 Construction noise
Pile driving involves using impact hammers to install large steel or concrete piles by driving them into the seabed to provide a solid structural foundation for construction. For floating wind farms, a different mooring system is used where anchors or piles are used. Fixed and floating wind farms therefore have different construction methods and different levels of noise emissions. One of the most significant effects associated with this activity is the production of high-intensity impulsive vibration which can have potentially multiple negative impacts on fish physiology (i.e. changes in heart rate, respiration and metabolism) and behaviour (i.e. avoidance and loss of group cohesion). The vibrational profile varies depending on the installation specifics, but pile driving has the capacity to produce very high sound pressure levels exceeding 250 dB re 1 μPa at 1 m recorded (Nedwell et al, 2007; OSPAR, 2009). Research shows that pile driving produces sound frequencies between 100 Hz and 2 kHz (Hawkins & Johnstone, 1978; Bailey et al., 2010) which coincides with the detection range of many fish species (see Table 6).
Potential impacts of pile driving vibration will be species specific, as different species will have different detection thresholds (Table 6 ). Interestingly, even closely related species such as Atlantic salmon and brown trout can differ considerably in threshold levels (see Figure 13). Parvin et al. (2007) suggested that lethal effects occur from peak-to-peak vibration levels exceeding 240 dB re 1 μPa and physical injury at noise levels of 220 dB re 1 μPa.
Potential barotrauma injuries caused by pile driving include; haematoma (in fins, body, swim bladder, gonads, muscle and organs), deflation of swim bladder, haemorrhage of organs, and laceration of swim bladder and organs (Halvorsen et al., 2012). These range from mild to lethal. In a study by Halvorsen et al. (2012), Chinook salmon (Oncorhynchus tshawytscha) were exposed to high energy impulsive sound similar to pile driving to find the threshold for injury onset, which is the level of sound exposure that causes a significant increase in the likelihood of injury. In this study, the threshold varied from 177 to 180 dB re 1 μPa.
Pile driving may also cause various behavioural effects that may lead to increased metabolic costs and mortality. Herbert-Read et al. (2017) showed that playbacks of pile driving sound lead to shoals of juvenile seabass (Dicentrarchus labraxI) becoming less cohesive and less directionally ordered. Mueller-Blenkle et al. (2010) studied the behaviour of sole (Solea solea) and cod (Gadus morhua), held in large net-pens, in response to pile driving playbacks. Both species showed increased swimming speed during playbacks and signs of moving away from the vibration, while cod also showed freezing behaviour at the start and end of the sound; these effects were happening even at relatively low received sound pressure levels (144 - 161 dB re 1 μPa). Although there are many examples of negative impacts of pile driving, one study showed no or very small effects: van der Knaap et al. (2022) showed that acoustically tagged free ranging Atlantic cod showed small movements away from the sound source but did not leave the study area.
Pile driving can potentially also have indirect impacts on fish physiology and behaviour by altering habitat quality. Impacts of pile driving are more intense at fixed wind farms than floating wind farms where different anchor types may be used (some of which do not require pile driving). Pile driving causes sediment disturbance which can alter habitats in addition to reducing water quality and oxygen availability. This in turn may lead to changes in the fish community structure. However, this potential impact tends to be short term and with a limited zone of influence, therefore not leading to a permanent change.
3.4.1.5 Operational noise
Although sound levels during construction are the highest and the most likely to cause negative impacts on surrounding animals, operational noise may also have negative effects on surrounding animals. Additionally, while construction noise is occasional and comparatively short-term, operational noise will be long-term and may continue for several decades, with typical wind farm consents being 30 years (Risch et al., 2023). Offshore wind turbines create noise through moving mechanical parts in the nacelle, wind induced vibration of the tower, and in the case of floating turbines, mooring lines and dynamic cables (Tougaard et al., 2020). This noise is transmitted to the water in two ways, through air and through the supporting structure. Noise level is related to wind speed, with higher wind speeds leading to louder noise levels. However, as an increase in wind speeds also leads to an increase in the ambient sound levels through wave action, therefore the relative contribution of operational noise, in relation to ambient sound may not be significantly higher (Westerberg, 1994). The ambient noise level will be determined by the topography and weather conditions of each site and therefore noise levels are likely to vary considerably between sites. Operational noise tends to be in the lower frequencies (< 1 kHz) and generally low intensity (Madsen et al., 2006; Risch et al., 2023). This overlaps with the hearing range of most of the 10 focal species (see Table 6); Atlantic salmon, brown trout, European eel, three-spined stickleback, sea lamprey and likely also European flounder, twaite shad and allis shad.
Degn (2000) analysed the underwater noise produced by offshore wind turbines at Danish and Swedish wind farms. They demonstrated that airborne noise contributed relatively little to the underwater noise. It was also found that the ‘noisiness’ of the foundation type varied with frequency; steel tube monopile foundations were noisier in the frequency range 50 - 500 Hz but concrete foundations were noisier at <50 Hz. For frequencies >1 kHz, the underwater noise from wind turbines was not greater than the ambient noise but it was higher when the frequency was <1 kHz. This report also showed that offshore wind farms do not produce ultrasound (frequencies > 20 kHz). Marmo et al. (2013) also found that different foundation types had different acoustic outputs. Westerberg (1994) measured operating noise levels of a wind farm in the Swedish coast and found noise increases of up to 20 dB over the ambient noise levels. At 300 meters, sound levels at 16.7 Hz were 5 dB above ambient noise levels. Tougaard et al. (2020) reviewed the literature on operational noise from wind farms and compiled available results. They found that for the 17 wind farms included in their study (which ranged in size from 0.2 to 6.15 MW), the dominant noise frequency varied from 25 to 400 Hz between sites and the estimated total sound pressure level varied from 81 to 137 dB re 1 μPA. Measurement distances ranged from 14 to 1000 meters. They also compared these values to sound pressure levels measured from ships at similar distances and found that for a given distance, the ship noise was at least 20 - 30 dB higher than the operational noise from turbines. When modelling sound levels with distance, wind speed and turbine size, they found that for all foundation types (concrete, monopile, jacket and tripod), there was a decrease in sound levels with distance but increase with wind speed and turbine size.
Operational noise from conventional and floating wind farms will have some differences, with the main difference being mooring-related noise. Risch et al. (2023) recorded operational noise at two floating wind farms in Scotland; one on a semi-submersible foundation and another on spar buoys. They found that noise was concentrated at frequencies below 200 Hz. They found that noise levels increased with increasing wind speed. At wind speeds of 15 m/s, the operational noise levels at the two farms were 148.8 dB 1 μPa and 145.4 dB 1 μPa respectively. They predicted that the sound levels (at 15 m/s wind speeds) remained above the ambient levels for maximum distances of 3 - 4 km from the centre of the wind farm. It should be noted however that these were small scale farms with 1-7 turbines.
There have been a limited number of studies looking at potential impacts of operational noise on fish populations. Westerberg (1994) found that catches of cod and roach (Rutilus rutilus) near a coastal wind turbine (within 100 m) increased during times when the turbine was stopped, which could suggest that these fish avoid the turbine during its operation when it is producing noise. Similarly, in a multi-year study Bergström et al. (2013) found that catches of eelpout (Zoerces viviparus) and European eel within a wind farm were lower during higher noise levels. Winter at al. (2010) however found no difference in the behaviour of tagged cod during low and high wind speeds which acted as a proxy for noise levels.
3.4.1.6 Vessel noise
Construction and maintenance of offshore wind farms will lead to an increase in vessel activity in an area. This will be particularly significant during construction but there will be an increase during the operational stage as well. Vessels used for construction are larger than for example many fishing vessels, and therefore may cause more noise. Noise from boats comes from multiple sources: engines, gear boxes and propellers which all produce slightly different frequencies of sound. Other stimuli associated with vessel movements may include visual cues, particle acceleration, ship bow wave and stimulated bioluminescence (De Robertis & Handegard, 2013; Mitson, 1995), however these will not be covered in this review. Vessel avoidance by fishes has been reviewed by De Robertis & Handegard (2013); typical reactions to moving vessels include diving and horizontal movements.
There are many examples of avoidance behaviours by fish in the presence of passing boats. This avoidance may be in response to visual or vibrational stimuli. At night, shoals of herring are found in the top 100 m of the water column and Vabø et al. (2002) found evidence of herring (Clupea harengus) moving away from a survey vessel during nighttime surveys. Another species that has well recorded avoidance behaviour to vessel noise is cod (Gadus morhua) (e.g. Handegard et al., 2003; Ona, 1988). There is some evidence that cod can detect and demonstrate avoidance behaviour of a trawling vessel over 2 km away (Buerkle, 1977).
For salmonids, Xie et al. (2008) showed that adult sockeye salmon (O. nerka) and pink salmon (O. gorbuscha) in a Canadian river moved away from a survey boat, but this effect seemed to be over a relatively short distance as it was only evident when the distance between the boat and fish was <7 m. However, they also saw evidence of other shoals of fish that were further away reacting to the avoidance behaviour of fish that were nearer to the boat. Thus, suggesting that vessel avoidance may lead to a small-scale behavioural cascading event. Van der Knaap et al. (2022) found that juvenile pink salmon and chum salmon (O. keta) responded to boat noise with typical anti-predatory behaviours such as increased swimming speeds, diving and forming tight schools.
The size, shape and speed of vessels will impact the extent that avoidance behaviour is exhibited. Fernandes et al. (2000) found that a research vessel built in a way to limit noise emission, did not lead to avoidance behaviour in herring, when compared with an autonomous underwater vehicle. However, the relationship between noise produced and boat design is not always clear; a study by Ona et al. (2007) compared the difference between a vessel designed to be ‘silent’ and a standard research vessel that conducted herring surveys using sonar. While the two vessels recorded similar densities of herring shoals (suggesting no difference in large-scale avoidance between the vessels), fish responded to the more silent vessel with a more intense and prolonged avoidance reaction (measured as the vertical mean swimming velocity). This highlights that even ‘silent’ vessels are not completely quiet and sound may not be the only significant stimuli to consider.
The potential impact of noise associated with increased boat traffic on diadromous fish at offshore wind farm sites during the construction, operation and maintenance may include avoidance behaviour. However, these potential impacts are unlikely to be significant as most fish will only be affected by vessels that are in close proximity. In addition to distance to sound source, the extent of the response will also depend on the level of the sound source. Additionally, the effects of boat noise will likely differ between species due to their behaviour (especially depth preference), detection sensitivity and life stage. The potential impacts from vessel noise are more likely during the construction phase when the amount of vessel traffic will be the highest.
3.4.1.7 General discussion
Although the study of anthropogenic impacts on fish has received much more focus recently and the methods have been improved over the last decade, it is still important to be critical and consider methodology carefully when comparing studies. Popper and Hastings (2009) discussed this in their review and made several recommendations. Studies should be careful to assess the interplay of pressure and acoustic particle motion as the sound stimulus – most studies so far have focused on the former, but there are many species that are more likely to use the latter as the main sensory cue. Additionally, extrapolating results from one species to another is not appropriate, as different species vary so much in their physiology and their ability to detect sound (e.g. see Figure 12 & Figure 13). However, as information on this topic is required, representative species that are found near offshore wind farms should be identified and prioritised for future work. While results would not be directly comparable between species, even if they are closely related, it would allow some level of generalisation with similar species (Popper et al., 2022b). Comparisons between different types of exposure (i.e. pile driving vs. seismic air gun) should be made carefully as well due to the very different features of the vibrations produced.
When comparing studies, it is important to remember that results from studies in the laboratory cannot necessarily be extrapolated to outcomes in the wild. Studies in tanks will lead to unnatural sound conditions which are not representative of what wild fish would experience. Additionally, care should be taken when assessing results from studies done in the wild but with caged fish. In many cases this methodology is chosen for practical reasons (the ability to observe the study animals and collect them after the study for assessment of physical injuries), however, especially when assessing behavioural reactions of caged fish, it should be remembered that the reactions (or lack of) of these individuals may not be representative of how wild, unrestricted fish may behave. This is particularly true in cases where fish are exposed to relatively loud noises likely to cause physical injury; in the wild, fish would be likely to escape the area before the noise reaches a level which is causing harm (Popper & Hastings, 2009).
In general, there should be improvements in the methodology used for studies of underwater sound and fish hearing (Popper & Hastings, 2009). This includes standardised measurements of sound and potential damage (physical or behavioural), use of expert fish pathologists for autopsies, and consideration of not just acute physical damage but also increased stress levels which may lead to further issues. Additionally, care should be taken to consider the timescales of observing potential impacts – in many cases the study animals are euthanised very quickly (within a few hours) but there is some evidence that physical injury may take days to develop. Also, considering detection (cf. hearing) loss (temporary threshold shift or a permanent threshold shift) as a variable would require longer term studies. Another current knowledge gap is how potential noise impacts vary with different life stages – logistical reasons have limited most work to studies on adults but it is feasible to assume that exposure and effects on adults and larval stages for example will be different.
3.4.1.8 Expert panel
The consensus from the expert panel supported the findings of the literature review in that despite much research on this field, there are still many large knowledge gaps on fish sound sensing abilities and potential impacts from anthropogenic sound and vibration. All experts agreed that due to the lack of empirical data on this topic, it is very difficult if not impossible to speculate what potential impacts of offshore developments might have on diadromous fish. Carefully designed, very specific scenarios would be required before reliable assessments can be made. It was noted that existing research has focused on certain groups and taxa and no research exists for many species, even those that have high conservation or economic value. The weaknesses of existing studies were discussed, particularly in relation to laboratory studies that have many issues mainly due to the issue of how sound waves move in small tanks and therefore it is unwise to use results from these studies for real-life scenarios. Similarly, ‘caged fish’ studies in the sea (where fish are kept in cages in the sea in the vicinity of a sound source so their behaviour can be observed or they can be sampled after the study) can be problematic as in these studies fish do not have the opportunity to behave naturally, for example, escaping the sound exposure, and therefore any results should be viewed critically. An important point highlighted by the expert panel was the role of particle motion which is very important (in addition to sound pressure), however until recently it has been absent from most studies. This is likely due to the challenges of measuring particle motion, which is logistically difficult and expensive but without relevant data, results of effects studies are likely to be erroneous.
3.4.1.9 Species-specific related research
3.4.1.9.1 Atlantic salmon
- Knudsen et al. (1992) – Awareness reactions and avoidance responses to sound in juvenile Atlantic salmon, Salmo salar L.: The effectiveness of using sound (including infrasound frequencies) to deter juvenile Atlantic salmon was tested, using frequencies between 5 and 150 Hz. Fish showed avoidance at lower frequencies (10 Hz) but not at 150 Hz.
- Harding et al. (2016) – Measurement of detection in the Atlantic salmon (Salmo salar) using auditory evoked potentials, and effects of pile driving playback on salmon behaviour and physiology: Wild smolts, hatchery smolts and hatchery adults were tested in laboratory conditions with frequencies of 100, 200, 300, 400, 500, 600, 700, 800 Hz. Fish responded to all tested frequencies and there were no differences in auditory evoked potential threshold levels between the three fish groups. In a second experiment, salmon were exposed to pile driving playback and its effect on behaviour and physiology was tested. The pile driving noise did not seem to result in observed behavioural differences between experimental and control conditions.
3.4.1.9.2 Brown trout
- Nedwell et al. (2006) – An investigation into the effects of underwater piling noise on salmonids: The potential impact of pile driving on farmed brown trout (used as a proxy for salmon) was tested through behavioural responses in five open water cages distributed at different distances. The pile driving unweighted Source Levels were 193 and 201 dB re 1 μPA at 1 m, noting the intensity but frequency was reported. In response to pile driving no startle reactions were observed and no internal barotrauma was found. An audiogram for brown trout found detection ranges of 20 - 1000 Hz but fish exhibited a higher threshold than the closely related Atlantic salmon.
3.4.1.9.3 European eel
- Sand et al. (2000) – Avoidance responses to infrasound in downstream migrating European silver eels, Anguilla anguilla: The effect of infrasound (frequency of 11.8 Hz) on migrating European eels was tested in a river. Infrasound exposure led to eels moving away from the sound source, showing that eels are able to detect very low frequencies.
- Deleau et al. (2020) – Use of acoustics to enhance the efficiency of physical screens designed to protect downstream moving European eel (Anguilla anguilla): Efficacy of acoustic stimuli to guide silver eels towards a bypass channel was tested and shown that this led to improved efficiency of physical screens. Treatments used continuous broadband sound of 60-1000 Hz and a pulsed sound of 100 Hz.
- Bergström et al. (2013b) – Study of the Fish Communities at Lillgrund Wind Farm: Final Report from the Monitoring Programme for Fish and Fisheries 2002–2010.: 300 eels were acoustically tagged and 100 provided useful information. Similar numbers of eels passed a transect at a wind farm during baseline and operational periods, however there was a difference in time taken to move past the wind farm between higher and lower production which was used as a potential proxy for sound). Migration times were longer during higher production, however as no sound measurements were done, it is not possible to determine a causation.
3.4.1.9.4 Three-spined stickleback
- Andersson et al. (2007) – Swimming behaviour of roach (Rutilus rutilus) and three-spined stickleback (Gasterosteus aculeatus) in response to wind power noise and single-tone frequencies: This study investigated how swimming behaviour of stickleback was influenced by offshore wind turbine noise in laboratory conditions. Fish were exposed to 25 Hz, 160 Hz, 200 Hz, and 500 Hz. Stickleback responded to the treatments with typical stress-related behaviours such as twitching, backward swimming and freezing.
- Purser & Radford (2011) – Acoustic noise induces attention shifts and reduces foraging performance in three-spined sticklebacks: The effect of acoustic noise on stickleback foraging performance was investigated in laboratory conditions. The addition of noise did not impact the total amount of food eaten but it increased food-handling errors (missed prey) and startle responses.
- Voellmy et al. (2014) – Assessing effects of increased noise levels on fish behaviour: Stickleback anti-predatory and foraging behaviour was investigated in laboratory conditions in response to anthropogenic (shipping) noise. Under additional noise, sticklebacks responded to a predatory stimulus sooner and consumed less Daphnia (caused by additional foraging errors).
3.4.1.9.5 River lamprey
- No in situ or laboratory experiments identified.
3.4.1.9.6 Sea lamprey
- Mickle et al. (2019) - Detection capabilities and behavioural response of sea lamprey (Petromyzon marinus) to low-frequency sounds: Using auditory evoked potentials, sea lamprey responded to frequencies of 50 - 300 Hz and in behavioural trials, a response (increase in breaching events and activity levels) was found between 50 - 200 Hz.
3.4.1.9.7 European flounder
- Maes et al. (2004) – Efficacy of an acoustic fish deterrent (AFD) at a nuclear power plant cooling water intake was tested. The AFD produced sound frequencies of 20 - 600 Hz. It was found that when the AFD was on, total catch of European flounder significantly reduced by 37.7 %, respectively.
3.4.1.9.8 European smelt/sparling
- Maes et al. (2004) – Efficacy of an acoustic fish deterrent (AFD) at a nuclear power plant cooling water intake. The AFD produced sound frequencies of 20 - 600 Hz. They found that when the AFD was on, total catch of European smelt and European flounder significantly reduced by 53.5 % and 37.7 %, respectively.
3.4.1.9.9 Allis shad
- No in situ or laboratory experiments identified.
3.4.1.9.10 Twaite shad
- Teague & Clough (2014) – Investigations into the response of 0+ twaite shad (Alosa fallax) to ultrasound and its potential as an entrainment deterrent: Wild-caught 0+ shad were tested in behavioural trials to see if avoidance could be elicited with ultrasound. The study animals showed startle responses to sound frequencies between 30 and 60 kHz, peaking around 45 kHz.
3.4.1.10 Authors’ assessment on the potential impact
Sound and vibration generated during the construction and operational stages has the potential to have a negative impact on all 10 focal species if they are exposed. The potential impacts are likely to be higher during construction than operation, as that is when higher intensity noise is present (pile driving). However, as operational noise will be much more long-term, it may also potentially have impacts. As the species represent very different evolutionarily strands it is not possible to consider them as a single group. The two shad species have the highest hearing range and they can hear ultrasound up to 60 kHz. For the other species that have studies available, hearing range seems to be between 20 – 600 Hz, with the European eel seemingly able to sense very low frequency infrasound. Much of the sound associated with construction and operation of wind farms is within the hearing range of the 10 focal species. Although it is reasonable to assume that all species could potentially be impacted, it is very difficult to estimate the magnitude of this potential impact. Much more research is required to provide this information. All conclusions are the authors’ opinions based on extrapolation from available evidence and therefore have been formed with low confidence.
3.4.1.11 Key evidence gaps / recommendations for future research
Despite considerable work on the topic of sound perception of fishes over the past 40 years or more, there are still significant evidence gaps remaining. Without understanding the relative contributions of sound pressure and particle motion to sound perception in fishes and the detection ranges and sensitivity of diadromous fish to sound (which should include thresholds for injury and displacement), it is not possible to fully evaluate the potential impact of sound from wind farms during construction and operation. To address the identified knowledge gaps, it is recommended that a combination of carefully planned laboratory and field studies are undertaken. Outlined below are a series of potential key evidence gaps and the appropriate methodology that would be required to answer each question.
3.4.1.11.1 The relative contribution of sound pressure and particle motion for sound perception
Most of the research undertaken on the potential impact of sound on fish behaviour and physiology has focused on measuring sound pressure, while the particle motion of sound perception has been largely unstudied. This is due to the financial and technological restraints of particle motion research, but there is a clear need for further understanding of the effects of sound on fish behaviour and therefore further research in this field should be prioritised. As sound transmission is very different in laboratory conditions compared with in the field, this work should be undertaken in the field whenever possible. It is recommended that an interdisciplinary approach is taken and in addition to fish biologists, a specialist in sound pressure and particle motion is consulted on the design of the study.
3.4.1.11.2 Improved knowledge on the detection ranges and sensitivity of diadromous fish
Despite decades of research on aquatic bioacoustics, there are still significant knowledge gaps in the sound detection capabilities of fishes. The lack of these data has been a challenge for estimating potential impacts of sound generated during the construction and operation of offshore wind farms.
A multi-method approach would be best for this study and both electrophysiological and behavioural responses should be investigated. It is highly recommended that studies are conducted on both juvenile and adult life stages. This work would require both laboratory studies investigating the hearing ranges of fishes and sensitivity to sounds and field studies investigating the likely behavioural responses of fishes to the sounds generated during operation. It is vital that the studies replicate as much as possible the sounds generated from offshore wind farms at different phases to make sure that measurements of responses to sound are made in realistic conditions. It is strongly recommended that an expert in acoustics is consulted on the design of the study.
3.4.1.11.3 Thresholds of injury and displacement
For many of the diadromous fish species, there is still uncertainty over the thresholds for injury and behavioural change (especially displacement) in response to construction and operational sound. There is also very little or no data available from studies conducted in realistic field conditions.
This study would be best answered by an acoustic telemetry study that has receiver coverage with the appropriate resolution to pick up very small scale (<100 m) movements. A grid array should be placed around or near an offshore renewables construction site. While information on diadromous fish would be most valuable, trying to ensure spatial and temporal overlap of the fish movements with construction activity would be challenging. Therefore, a suitable study species would be a species known to occur within the development, such as cod. Telemetry data should be combined with detailed records of construction activity and sound and vibration measurements.
3.4.1.11.4 Mitigation against construction noise
There is limited direct evidence of the potential impacts of anthropogenic noise on diadromous fish currently, however it is likely that especially some construction activities such as pile driving will have an impact at close enough distance, and therefore investigating potential mitigation methods is important. While operational noise during standard wind conditions is mostly considered to be within background noise levels (Tougaard et al., 2020), noise levels during construction have potential to exceed tolerance levels and have potentially negative impacts on fishes in the vicinity – however it is also likely that free-swimming fish will move away from a sound source as they initially detect it. Mitigation against the noise, especially pile driving, can be done (for example in the form of a bubble curtain; Nehls et al., 2016; Stokes et al., 2010) but there is uncertainty about the efficacy of these methods: a review of noise abatement systems for offshore wind farm construction noise was compiled by Verfuss et al. (2019). Additionally, almost all of the work on mitigation methods has focused on marine mammals. Therefore, we highly recommend the efficiency of mitigation methods are investigated using both laboratory and field studies. In laboratory studies, it is crucial that the simulated conditions are as similar as possible to what would be experienced in the field, which are very difficult to achieve, therefore, realistic measurements of sound generated during construction should be taken in the field.
We recommend identifying 2-4 offshore wind farm development sites where construction is scheduled to begin (and there are plans for using noise mitigating methods) and developing a collaborative survey programme with the developers. Standardised recordings are to be taken at each site with and without mitigation methods for the duration of each pile driving event. Appropriate replication is required and therefore multiple recordings at each farm need to be taken.
3.4.1.11.5 General notes
Spatial and temporal scales of data collection: For many of the evidence gaps highlighted in this section, laboratory studies are likely to be unable to replicate natural field conditions, and therefore field studies should be preferred. However, laboratory-based studies may be useful for certain topics such as testing particle motion equipment – in these cases the studies need to be replicated with large enough sample sizes. Field studies require replication between sites and over long enough time periods to account for site-specific differences (depth, substrate type etc.) and variations in environmental conditions (wind, temperature etc.).
Feasibility and challenges: There are many knowledge gaps in this field despite decades of research which highlight the complexity of the topic. It is important that all studies, whether in the laboratory or in the field, replicate realistic sound conditions that the fishes would experience, most importantly during construction. Due to the difficulties of replicating natural sound scenarios in a laboratory, field-based studies are recommended. There will be technological challenges, especially with measuring particle motion, including for example calibration of the machinery. It is highly recommended that experts in this field are consulted during the design and implementation of studies to answer the above questions. Each question has its own challenges in designing a study requiring careful consideration. For example, if using acoustic telemetry to investigate the threshold and displacement responses of fishes to noise from wind farms, a future study is highly dependent on; (1) what species are likely to already be present in the area; (2) species availability and capture efficiency; (3) lack of existing community structure and behavioural baseline data prior to the development of a wind farm making it difficult to distinguish the motivation (natural vs impacted) behind observed responses. However, a carefully planned study could account for most of these challenges.
3.4.2 Changes in Light Patterns from Turbine Blades
Shadow flicker has been defined as “Under certain combinations of geographical position, time of day and time of year, the sun may pass behind the rotor and cast a shadow over neighbouring properties. When the blades rotate, the shadow flicks on and off; the effect of impact is known as ‘shadow flicker’.” (ClimateXChange, 2015). This effect will be the same for onshore and offshore turbines, however if and how this is perceived under water should be investigated. Another potential impact related to light pattern change from offshore wind farms is the secondary effect of light reflection from turbine structure surface. Potential impacts of shadow flicker and light reflection will be relevant to the photic zone of the water column and impacts may be most significant in the surface layers.
Potential impacts from changes in light patterns from turbine blades on fish is a very understudied field. Current research on shadow flicker effects from turbine blades on Atlantic salmon was reviewed by Dodd and Briers (2021) who investigated the evidence base for shadow flicker effects and how this may impact the different stages of Atlantic salmon in freshwater. As part of this review, the authors could not find any studies that specifically addressed shadow flicker effects on Atlantic salmon or other fish species.
While there is some information available about the response of fish species to changes in light intensity (e.g. responses to strobe light or artificial light at night), there is no published information that this review could identify about the responses (biological or behavioural) of any fish species to artificial light patterns which are associated with shadow flicker.
Although there is a lack of direct studies, it is plausible that turbine blade shadow flicker may have a potentially negative impact on fish at offshore wind farm sites. One potential impact could be avoidance behaviour due to the flicker. Although not directly comparable, there have been several studies in salmonids (Fjeldstad et al., 2018; Jesus et al., 2019) and other species (i.e. American eel: Patrick et al., 2001; sea lamprey: Johnson et al., 2019) showing that artificial light flicker in the form of strobe light can be successfully used as a deterrent preventing fish passage. Aronsuu et al. (2015) found that up- and downstream migrating river lamprey stopped moving when faced with illuminated bridges. Therefore, fish may seek to avoid shadow flicker around offshore wind farms. Another possible negative effect of shadow flicker is its potential impact on predator avoidance, especially in relation to avian predators. This effect may be similar to turbidity, which reduces a fish’s perceived predation risk (Gregory, 1993). If the shadow flicker is constant, it is possible that there may be a level of habituation and the perceived risk and stress would decrease over time.
3.4.2.1 Expert panel
This was the smallest expert panel (with two external experts), as due to the lack of research on this topic it was difficult to identify suitable candidates. However, the workshop had a good discussion. It was a clear consensus of the panel that of all topics, this one was the most understudied and the panel was not aware of any research on the topic either. The different forms of light pattern changes were discussed, and it was highlighted that in addition to shadow flicker, it is important to consider light reflection of the surfaces of the turbine. It was noted that this topic requires an interdisciplinary approach and physicists are required in the process of defining appropriate effects of the physical process. Although species that use the top layer of the water column may be most likely to be impacted, it was noted by one expert that shadow flicker will be visible through the photic zone which may be down to 200 m, although 100 m is more likely. The panel had a long discussion on the likelihood of habituation by fish to light pattern changes. This would be impacted by exposure time and first reaction; for example, if an individual quickly moves away after first exposure there will be no opportunity for habituation. This is likely to also vary between migratory and sedentary fish, with migratory fish much less likely to habituate. The potential magnitude of the impact may be related to if a fish is exposed to the impact at the edge of the wind farm or if it enters the development in which case the stimulus would be multiplied and fish may be disoriented. In relation to this, fish may be less likely to detect a wind farm as they approach it (cf. birds), therefore the likelihood of entering a farm could be high. It was discussed if the shape of a wind farm might relate to the likelihood of fish avoiding or being able to exit and it was suggested that a long and thin array might be the best. When considering the actual potential impacts of light pattern changes, a key potential impact of shadow flicker is that it could be confused as a predator cue; responses to this are likely to be different for benthic and pelagic species.
3.4.2.2. Species-specific accounts
3.4.2.2.1 Atlantic salmon
- No in situ or laboratory experiments identified.
3.4.2.2.2 Brown trout
- No in situ or laboratory experiments identified.
3.4.2.2.3 European eel
- No in situ or laboratory experiments identified.
3.4.2.2.4 Three-spined stickleback
- No in situ or laboratory experiments identified.
3.4.2.2.5 River lamprey
- No in situ or laboratory experiments identified.
3.4.2.2.6 Sea lamprey
- No in situ or laboratory experiments identified.
3.4.2.2.7 European flounder
- No in situ or laboratory experiments identified.
3.4.2.2.8 European smelt/sparling
- No in situ or laboratory experiments identified.
3.4.2.2.9 Allis shad
- No in situ or laboratory experiments identified.
3.4.2.2.10 Twaite shad
- No in situ or laboratory experiments identified.
3.4.2.3 Authors’ assessment on the potential impact
There is very little research done on this specific topic and none addressing shadow flicker directly, therefore it is very difficult to make an assessment of the likelihood and significance of potential impacts of turbine shadow flicker and light reflection. The potential impact is likely to be very localised geographically, but it is not possible to assess the potential impacts and which species may be most likely to be affected. Based on extrapolation of the available evidence and the authors' opinion (formed with low confidence due to the lack of available information), there is not sufficient evidence to support or refute any potential impact of shadow flicker or light reflection on diadromous fish species around offshore wind farms. As such all conclusions are the authors’ opinions based on extrapolation from available evidence and have been formed with low confidence.
3.4.2.4 Key evidence gaps / recommendations for future research
Operation of wind farms will lead to light pattern changes, including shadow flicker resulting from both the movements of the turbine blades and the reflection of sunlight through the turbine structure. Light pattern changes may lead to behavioural and physiological changes for fish and other marine animals. While some aspects of the potential impact of light pattern changes have received much attention, such as artificial light, most aspects have received little or no attention. Currently no research exists, that we could identify, on the potential impact of shadow flicker on the behaviours of fishes.
To address the identified knowledge gaps, it is recommended that a combination of laboratory and field studies are conducted. Outlined below are a series of sub-questions and the appropriate methodologies that would be required to answer each evidence gap
3.4.2.4.1 Laboratory study on the effects of light pattern changes
Some of the potential impacts of shadow flicker may include avoidance, physiological stress and reduced ability to avoid predation (especially avian). These topics should first be addressed in a laboratory setting, either an artificial flume or a mesocosm, where conditions can be controlled. Both constant shadow flicker and light reflection should be investigated. These conditions would be artificially created above the water column and then behavioural responses by fish quantified, under different treatments. We recommend three studies: investigation if fish will show avoidance when faced with changing light conditions (ideally in an artificial flume); will light pattern changes lead to increased stress (ideally using a respirometry set up); and will predator avoidance be reduced with light pattern changes (ideally in an artificial flume with simulated predators).
As a secondary aim, studying the potential habituation of fish to constant shadow flicker would be beneficial. This would have two parts; firstly, if a fish is initially stressed by the presence of light pattern changes, will it eventually habituate with ultimately negative potential impacts detected after a period of time, and secondly, could habituation to shadow flicker lead to a scenario where there is no response to similar stimuli (i.e. avian predator) in the same or another context.
3.4.2.4.2 Field study on the effects of light pattern changes
Ideally controlled and replicated laboratory studies would be followed by field studies so the potential effects could be studied in real life scenarios. A fine scale acoustic telemetry set up could be used to study movement patterns of fish around the edges of wind farms where they will be exposed to both natural conditions and light changes caused by the turbines. Video camera arrays could also be deployed for detailed behavioural analysis in specific locations.
Spatial and temporal scales of data collection: Appropriate replication is needed for all laboratory experiments and field studies, including within and between sites comparisons for the field studies.
General Notes
Feasibility and challenges: The laboratory study designs are straightforward and allow for careful control and replication. However, a laboratory study would be limited in the number of species and their different life stages that would be available to research. For example, due to the current low numbers, it may be challenging to capture the two shad species and European smelt. Additionally, it is not known how well especially the adults of these species will adapt to laboratory conditions. For salmonids, conducting experiments on parr would be the most feasible option, but conclusions would have to be drawn regarding the responses of later life stages (smolts and adults) that undertake the migration and are exposed to real light changing effects.
All ten focal fish species should be investigated, but especially the three species that are a qualifying feature of SACs in Scotland. Logistically, work may be limited to those species that are known to do well in laboratory conditions (i.e. salmonids, European eel, lampreys, stickleback and flounder).
3.4.3 Electromagnetic Fields
Offshore wind farms transfer energy to the mainland power grid by subsea cables, including high voltage alternating current (HVAC) or high voltage direct current (HVDC) cables. The cable type will influence which characteristics of the magnetic field are emitted; HVACs emit a time-varying magnetic field, while HVDCs emit a static magnetic field (Gill et al., 2014). Shielding on the cables will stop the electric field from propagating to the environment but the magnetic field is not shielded. In both the AC and DC scenario, an induced electric field is created as fish and water currents move through the magnetic field. Additionally, HVACs create a magnetic field through rotation in the magnetic emission (Gill & Desender, 2020).
Offshore wind farms have inter-array cables that connect the turbines within a wind farm to each other and the hubs, and export cables that connect the offshore farm to the mainland. Inter-array cables are usually placed on or in the seabed, however floating wind farms use dynamic cables which are partly suspended in the water column. To date, HVACs have been typically used for distances of 15 - 50 km from shore and HVDCs for longer distances (Soares-Ramos et al., 2020). While HVAC cables are currently more common for offshore wind farms, HVDC cables are expected to be used more in the future as distances from the shore and power production increase (Soares-Ramos et al., 2020). These cables are usually made from a high-strength conductor surrounded by layers of insulation and protective materials.
Measuring the magnetic and electric fields emitted by subsea cables can be logistically challenging, especially for the induced electric fields, and this is likely one reason why there are limited numbers of measurements available. Some monitoring has been done however and most measurements are within the 1 to 100 μV/cm range (Table 7) (Gill & Desender, 2020; Hutchison et al., 2021). The Earth’s magnetic field varies from 25 to 65 μTesla from the equator to the poles and is the primary source of natural EMFs. Natural electric fields, known as bioelectric fields, are produced within organisms as a result of different biological processes (Bedore and Kajiura, 2013). HVDCs produce a static magnetic field while in HVACs it generally is a low-frequency sinusoidal field (Gill & Desender, 2020) near the cable which may interfere with cues that animals use based on the Earth’s electromagnetic field, and therefore can potentially impact animals which encounter them.
How species will react to EMFs will depend on their sensitivity to electric and magnetic fields. Species can be either electro-receptive, magneto-receptive or both. Electro-receptive species may use natural electromagnetic fields for migration (short or long distance), for prey (or predator) detection, and or identification of feeding or breeding grounds (Naisbett-Jones & Lohmann, 2022). Much of the electro-reception work has been done on elasmobranchs. Electroreception is an ancient trait thought to have appeared more than 500 million years ago but it has also evolved multiple times throughout teleost evolution (Alves-Gomez, 2001). It is widely found and best studied within chondrichthyans (Newton et al., 2021). Electroreceptive species are capable of detecting weak electric fields, which allows them to sense their surroundings. This is particularly useful for predator-prey interactions and especially in dark or murky environments where visual cues might be limited (Newton et al., 2021). Many species use electroreception for prey detection as this allows them to detect prey when camouflaged or hidden. In the same way, prey species will also use electric fields to detect the presence of a predator, giving them more time to react and escape. Considering how ecologically important electroreception is for many fish species, any anthropogenic changes to natural electric fields may have significant consequences (Newton et al., 2021).
Magneto-receptive species use the Earth’s magnetic field for orientation and navigation for short and long distance migration (Formicki et al., 2019). Magnetoreception is phylogenetically widespread amongst fishes, suggesting that it has either evolved multiple times or been heavily conserved through evolution - highlighting its importance (Naisbett-Jones & Lohmann, 2022). It has been suggested that animals can detect the magnetic field in two ways; either their internal compass is based on the polarity or the inclination of the magnetic field (Wiltschko & Wiltschko, 1972; Light et al., 1993; Lohman et al., 1995; Kimchi & Terkel, 2001). The focal species in this review that have been confirmed to use magnetoreception for migration include the Atlantic salmon (Putman et al., 2014), European eel (Cresci et al., 2017; Cresci et al., 2022) and anadromous brown trout (Formicki et al., 2004), and therefore these diadromous species may be most affected. However, it is likely that most if not all of the 10 species use magnetoreception to some extent (Naisbett-Jones & Lohmann, 2022). Much is still unknown about the mechanism and physiological basis of magnetoreception in fishes. However, it has been suggested that in bony fish (which includes all focal species except the two lamprey species in this report), magnetoreception may be achieved through one of three hypotheses: biogenic magnetite coupled to mechanoreceptors, electromagnetic induction, or series of biochemical reactions modulated by earth-strength magnetic fields (Naisbett-Jones & Lohmann, 2022).
There are a variety of potential impacts from EMFs on marine organisms, including behavioural (altered avoidance and attraction behaviours) and physiological (modified hormone levels: Lerchl et al., 1998). However, there is still a lack of studies on the potential impacts of anthropogenic EMFs that provide real clarity on realistic effects. As stated by Gill and Desender (2020), the potential impacts will vary depending on several factors which include the type of current, power level transmitted, cable characteristics, surrounding environmental factors and the specific species biology. While cables are buried into the substrate or laid on the seabed with protection (concrete mattressing, boulder placement, tubular protection), due to the intensity of the magnetic field, this could still have a potential impact if the animal encountering the cable is in close enough vicinity to the source. Burying or covering the cable will not reduce the level of electromagnetic fields, but it does increase the distance between the cable and animals and thus, may reduce the potential encounter by preventing the animal getting close to the source (Hutchison et al., 2021a). As cable burial depth is dependent on the seabed substrate and potential risks to the cable (for example from fishing), it is likely the burial depth in addition to the level of protection will vary along the cable (Hutchison et al., 2021a). In addition to considering the distance between a cable and an animal, it is also important to consider the operational power level as it is the combination of these two factors which will determine the EMF level experienced by the animal (Hutchison et al., 2020).
Hutchison et al. (2021a) modelled the influence of cable properties of a HVDC transmission cable. This model was based on the Cross Sound Cable that is in Long Island Sound, USA, which has a highest nominal current of 1175 A (330 MW, 300 kV). They found that as the separation distance of two bundled cables increased, the magnitude of DC magnetic field (DC-MF) positive and negative deviation increased, and that the positive and negative deviations were asymmetrical. Additionally, as the cable burial depth increased, the magnitude of DC-MF at the seabed surface decreased due to increased distance from source.
However, the literature suggests that due to the relatively low strength of EMFs from cables, it is unlikely that they have ecologically significant effects on fish (Gill & Desender, 2022), at least in terms of physiological effects. Nervous, cardiovascular, reproductive and immune systems could potentially be impacted by chronic exposure (Riefolo et al., 2016), however for most mobile species, chronic exposure is unlikely. When considering the potential impacts of EMFs on fish, it is important to take into account the frequency of encounters with one or multiple cables and therefore the potential cumulative effects (Hutchison et al., 2021a).
Cable |
EMF Measurements |
||||||
---|---|---|---|---|---|---|---|
Cable and location |
Specifications |
Type |
Method |
Magnetic field |
Electric field |
Spatial extent |
Reference |
Belgian OSW farms (Preliminary use of SEMLA device) Use: OSW inter-array (C-Power) and export cable (Northwind) Position: both buried |
Inter-array: not powered Export: 70 A |
AC |
Platform: vessel towed/suspended Swedish Electromagnetic Low-noise Apparatus ‘SEMLA’ (sledge). Measured: electric and magnetic fields, 3D. Position: on the seabed (magnetic sensor, 0.15m above seabed, electric sensors 0.52-1.04m above seabed). |
Max: 4 nT inter-array cable (OSW not operational; device suspended) Max: 17 nT export (at 15 m distance) |
Max: 0.3 mV/m inter-array (not operational) Max: 1.5 m/V export (at 15 m distance) |
n/a 10’s m |
1 |
Cable near the Naval Surface Warfare Centre, South Florida Ocean Measurement Facility, South Florida, USA* Use: naval test site Position: buried |
2-2.4 A, 0.98-1.59 A, 60 Hz |
DC AC |
Platform: AUV towed device Measured: magnetic fields, 3D. Position: 2.2 m above seabed. Measured: electric fields, 3D. Position: 4 m above seabed. |
Powered: Max 150 µT positive deviation, -50 µT negative deviation from ambient. Not powered: Mean 30 nT above ambient n/a |
n/a Powered: 60µV/m Mean 32 µV/m. Not powered: 10 µV/m |
~10’s m (estimated) ~150 m (estimated) |
2 |
Trans Bay Cable (85 km), San Francisco Bay, California, USA** Use: domestic Position: buried |
Max rating: 200 kV, 400 MW (variable power during survey) |
DC |
Platform: vessel towed drop-down device. Measured: magnetic field. Position: Surface tow (c.a. 14 m above seabed) and deep tow (c.a. 8 m above seabed). |
Surface tow: mean 117.0 nT (sd = 22.1) Deep tow: mean 300.5 nT (sd = 130.5) |
n/a |
~80 m (40 m either side of cable) |
3 |
Basslink (290 km), Bass Strait, Tasmania, Australia Use: state transfer Position: buried |
592 A, 237 MW (1500 A, 600 MW) |
DC |
Platform: vessel towed drop down device. Measured: magnetic field, 2D. Position: 5, 10, 15, 20 m above seabed. |
Range: 57.2 – 61.5 µT (background 61.6 µT) At 5 m height: 57.9 µT (background, 58.3 µT) |
n/a At 5m: 5.8 µV/m*** |
up to 20 m from seabed & 10-15m either side of cable horizontally |
4 |
Cross Sound Cable (40 km), Connecticut, USA Use: domestic Position: buried |
0-345 A (300 kV, 330 MW) |
DC |
Platform: vessel towed Swedish Electromagnetic Low-noise Apparatus ‘SEMLA’ (sledge). Measured: electric & magnetic fields, 3D. Position: on the seabed (magnetic sensor, 0.15m above seabed, electric sensors 0.52-1.04m above seabed). |
DC: 0.4-18.7 µT (expected) AC: max 0.15 µT (unexpected) (background, 51.3 µT) |
n/a AC: max 0.7 mV/m |
Magnetic fields: 5-10m. Electric field: up to 100 m (either side) |
5,6 |
Neptune Cable (105 km), New Jersey, USA Use: domestic Position: buried |
500 kV, 660 MW |
DC |
As above |
DC: 1.3-20.7 µT (expected) AC: max 0.04µT (unexpected) |
n/a AC: max 0.4 mV/m |
Magnetic fields: 5-10m. Electric field: up to 100 m (either side) |
5,6 |
BIWF Sea2shore (32 km), Rhode Island, USA Use: OWF export Position: buried |
502 A, 30 MW |
DC |
As above |
AC: 0.005 - 3.0 µT |
AC: 0.02 - 0.25 mV/m |
Up to 100 m either side of cable |
6 |
*Magnetic and electric field measuring devices were towed independently while the cable was powered and unpowered with AC or DC currents. **Mean anomalies accounting for total range for positive and negative deviations, in absence of bridges. ***Motionally induced electric field arising from water movement through the measured magnetic field, calculated at 0.1m/s water flow. References: 1. Thomsen et al., 2015; 2. Dhanak et al., 2015; 3. Kavet et al., 2016 & supp. Material; 4. Sherwood et al., 2016, 5. Hutchison et al., 2020; 6. Hutchison et al., 2018.
A particular concern about EMFs in relation to diadromous fish is the potential impact on migration routes and the concern that EMF exposure from cables may make it difficult for them to decipher migratory cues. Other potential consequences may be the potential for migration delays due to the avoidance of cables or disruption to the ability to sense natural geomagnetic cues. For this topic, it is important to consider both the inter-array cables and the export cables. While some species may be unlikely to overlap with offshore wind farms due to their fairly distant location, they may be much more likely to come across export cables in the coastal zone.
There have been very few field studies investigating this topic. For salmonids, a few studies exist, with two studies using Chinook salmon in San Francisco Bay. From work by Klimley et al. (2017) and Wyman et al. (2018) it was found that the magnetic anomaly from road bridges was an order of magnitude higher than that coming from the underwater cable; they found around 50% of the tagged Chinook salmon smolts in this study migrated past the bridges, thus, at least for fish which successfully passed the road bridges, the EMFs produced did not seem to have a significant impact on migration. For those fish that did not move past, it is not possible to say that this effect was purely due to the bridges, as this could also include natural mortality due to predation amongst other reasons. There was no significant difference in the proportion of successful migrants that entered the ocean before and after a HVDC cable installation. However, they found evidence of smolts moving away from the most direct route out to the open ocean and being misdirected towards the cable. In a laboratory study, Armstrong et al. (2015) investigated the behaviour of Atlantic salmon smolts in response to 50 Hz magnetic fields and found that there were no differences between the treatment and control trials. However, it should be considered that this study used Helmholtz coils that may not accurately reflect real EMFs and migratory species tested in laboratory conditions may not behave naturally.
Field studies on the effects of diadromous fish behaviour around power cables have also been conducted on European eel from studies by Westerberg and colleagues in Swedish waters. Westerberg & Lagenfelt (2008) used acoustic telemetry to study the behaviour of migrating adult eels as they moved over a subsea cable. The cable did not seem to be a barrier as eels approached and crossed the cable but there was evidence that the eels moved slower when they were closer to the cable in comparison to either before or after the cable. However, the spatial resolution of the study was not fine scale and therefore it is possible that smaller behavioural changes were missed, also it is possible that other factors such as water currents may have impacted the findings. Furthermore, no direct measurements of the EMFs were made and therefore a specific link to EMFs is not clear. In a laboratory study, Orpwood et al. (2015) studied the effect of an AC magnetic field of approximately 9.6 µT on the behaviour of European silver eels and found no impact of the treatment on the passage rate of eels. In a field study by Dunlop et al. (2016), the effect of an underwater power cable on a fish community was studied in the Great Lakes, it was noted that American eels (Anguilla rostrata) utilised the boulder substrate placed on top of the cable, suggesting that the eels were not deterred by any EMFs. However, in this study, no measurements of the EMFs were taken and the eels were not in a migratory phase. Hutchison et al. (2021) conducted a study on a HVDC cable (the Cross Sound Cable in US) which incorporated measurements of the EMF emissions using a bespoke sensor system and two years of acoustic tracking of American eels to study their behaviour in the vicinity of the cable. In 2018 the cable was not operational but in 2019 the cable was operating and eel responses to the EMFs could be studied; eels were detected passing the array during varying power outputs (0-229 MW). The cable was not an acute barrier to migration, however the eels did respond to the DC magnetic field (the maximal DC magnetic field deviation was 7.5 µT); as the positive anomalies increased, their mean step length (as modelled by Hidden Markov Modelling) increased, suggesting a behavioural change.
As swimming capabilities of juvenile fish are poorer compared to adults, they may potentially be more impacted by EMFs from cables if they encounter them (Cresci et al., 2022). Eggs and larvae are also likely to be more impacted by EMFs if they are exposed to them due to their early developmental processes (Gill & Desender, 2020) and the reliance on natural EMFs may vary at different life stages. Fey et al. (2019) studied the potential impacts of static DC magnetic field (10 mT) and electromagnetic field (1 mT) on eggs and larvae of rainbow trout (Oncorhynchus mykiss) and found that there were no effects on mortality, hatching time, growth or swim-up time, however the yolk sac absorption rate increased. In a similar study by Stankevičiūtė et al. (2019), eggs of rainbow trout were exposed to EMF (1 mT) for 40 days which led to alteration in the number of cell nuclei and nuclear abnormalities. This sort of long term exposure is only likely to potentially impact eggs and mostly sessile larvae, whereas older life stages would be unlikely to remain within the vicinity of power cables this long. Formicki et al. (2004) tested the swimming direction of brown trout larvae and fry in response to constant magnetic field and found that even as newly hatched larvae, brown trout are sensitive to magnetic field changes with most larvae and fry found to swim towards experimental chambers equipped with magnets. Considering that all but one of the 10 focal species spawn either in freshwater or the Sargasso Sea, and therefore are far from export cables, their likely exposure to EMFs during this life stage is very unlikely. However, where export cables enter estuaries and lower rivers there is a potential for some species that have their spawning and nursery habitat in the lower reaches of rivers. It is important to remember however that in freshwater, the electric- and induced electric-fields do not propagate.
Another possible impact of HVDC/HVAC cables is their ability to slightly heat up the surrounding sediment (if buried), although the evidence for this is still limited. This heat emission is higher in HVAC cables (Taormina et al., 2018). Heat emission could possibly have an impact on marine benthic spawning areas if they overlap. There is some suggestion that the predicted increase in heat would be small (1 - 2 °C) (NorthConnect) and the spatial extent of this effect would be very small, however other evidence suggests that the increase in temperature may be >10°C up to 40 cm from the cable (Emeana et al., 2016). However, as noted above, nine out of the 10 focal species of this review spawn either in freshwater, likely far away from grid connection points or the Sargasso Sea, and therefore their spawning areas are not in the vicinity of offshore cables. The only species which may be affected is the flounder. Heating of surrounding sediment may also potentially lead to changes in the benthic community composition around the cable but as the spatial extent of this potential impact is likely to be small (likely <1 m from the cable), it is unlikely that this will be a significant effect. However, it is possible that in the future these power cables may be brought ashore through large rivers and thus it is important to consider potential effects on eggs and juveniles in freshwater habitats.
The probability of exposure to EMFs is likely related to the biology and behaviour of the species, particularly their distribution in the water column. Benthic species, such as European flounder, are more likely to encounter underwater power cables due to their physical proximity to them if cable routes and their habitat overlap (Cresci et al., 2022; Hutchison et al., 2020). However, there are also examples of studies where no difference was found in flatfish numbers between cable and control sites. van Hal et al. (2022) conducted a study of paired trawls (one directly over the cable and one 500 m away from the cable) and found no difference in the number of three flatfish species (plaice, sole and dab) between the reference and control areas. However, the authors of this study state that their study had some flaws such as a relatively small sample size of 24 pairs of trawls. Migratory pelagic species may come into contact with the EMF emissions from dynamic cables associated with floating wind farms that are found in the water column however they are not well characterised to date.
3.4.3.1 Expert panel
Members of the expert panel agreed with the findings of the literature review in that this topic has been understudied currently and there is a clear need especially for more field studies to collect empirical data. Much of the existing relevant work has been done on elasmobranchs, with emerging research on different life-stages of invertebrates and fish, and much less is available for the 10 fish species that this review focuses on. This means that a level of extrapolation about the general effects will be required. It was highlighted by the expert panel that it is very important to consider potential impacts on different life stages for the different species, as these may differ significantly and effects during early development may have long lasting consequences. There is also a clear need to consider potential impacts at a population level, which is lacking in the existing research. Additionally, when designing and conducting studies it is important to carefully consider what an ‘impact’ is; what is the threshold for considering it a negative effect on the fish that comes across the stimuli and is exposed to it. Fish may sense a change in the EMFs as they encounter cables, but whether or not this will always lead to a physiological or a behaviour response needs to be determined. It was also noted that it is important to account for cumulative effects, particularly with regards to increased energy expenditure through avoidance. This is mostly related to species that may come across with cables multiple times and likely not applicable to all focal species in this report. It was noted that behavioural change may be a common response to fish coming across EMFs and therefore any diversions could lead to considerable energy expenditure. This is also why considering encounter rate is important as repeated exposure is likely to have bigger impacts but at the same time, fish may pass developments without actually encountering EMFs. Encounter rate should also be considered to provide context for the studies presently available, as the realistic encounter rates with EMFs are low and therefore this may influence the implications of these studies. Although most of the potential impacts may take place in the marine environment, cabling in freshwaters as part of the cabling infrastructure will need to be considered and impact assessments may need to consider potential impacts on freshwater life stages as well. In addition to focusing on long-term migrations that most of the focal species undertake, it is also important to consider shorter, daily migrations that may be affected by EMFs. Another key point highlighted by the expert panel discussions was that whether a species can (a) detect EMFs and (b) will they be impacted, are two different questions – therefore future work needs to design studies in a way that can separate these two questions. Interpreting existing research needs to be done critically, as both field and laboratory studies have weaknesses but equally both have merit for specific questions. Field-based telemetry studies investigating the potential impacts of EMFs may sometimes lack controls and therefore it can be difficult to assign a behavioural change to one specific cause when there are so many other variables that could have caused an effect. However, this can be avoided by a detailed study plan that includes controls. Laboratory studies provide the opportunity for controlled experimental design and fine scale measurements, but it is more difficult to replicate natural conditions and the added stress of a wild fish being confined in a tank may influence behaviour. Therefore, for any new study, care should be taken to determine whether a laboratory or a field study would provide the best methodology for providing most accurate results. Future work should also be interdisciplinary, and physicists should be included to ensure that measurements of EMFs are undertaken and done accurately. Due to the relative lack of research, there are many open questions still; How do EMFs vary over time and space? How do animals respond to them? During migration, what level of exposure is a problem? What is the encounter rate?
3.4.3.2 Species-specific related research
3.4.3.2.1 Atlantic salmon
- Poddubny (1967) – Poddubny et al. (1979): demonstrated milling behaviour and a time delay for salmon, Salmo salar L., passing under overhead AC power lines in a river.” Op. Cite.
- Armstrong et al. (2015) – Scottish Marine and Freshwater Science Vol 6 No 9: Behavioural Responses of Atlantic Salmon to Mains Frequency Magnetic Fields. [technical report]: Post-smolts and adult Atlantic salmon were tested in laboratory conditions (large arena) for their behaviour in response to 50 Hz magnetic fields as they moved through Helmholtz coils. There were no significant differences in the approach and departure times when the coils were activated and when they were not.
3.4.3.2.2 Brown trout
- Formicki, et al. (2004) – Behaviour of trout (Salmo trutta L.) larvae and fry in a constant magnetic field. Journal of Applied Ichthyology, 20, 290-294: Larval and fry responses to different magnetic fields were tested in a lab and it was found that even at this early development stage, both were responding to the magnetic anomalies and chose the chambers where the entrance showed a magnetic field intensity higher than that of the Earth’s magnetic field.
3.4.3.2.3 European eel
- Westerberg & Lagenfelt (2008) – Sub-sea power cables and their migration behaviour of the European eel: The effect of a 130 kV AC power cable on migrating eel behaviour was tested in the Baltic sea. With a sample size of 60 tagged eels, it was shown that the swimming speed of the eels was significantly slower when crossing over the sub-sea cable, in comparison with a nearby area. However only 2 out of 60 eels did not migrate over the cable.
- Westerberg & Begout-Anras (2000) – Orientation of silver eel (Anguilla anguilla) in a disturbed geomagnetic field. In: A. Moore and I. Russell (eds.) Advances in Fish Telemetry. Proceedings of the 3rd Conference on Fish Telemetry. Lowestoft: CEFAS, pp. 149-158: Orientation of silver eels was studied in the presence of a submarine HVDC power cable. Approximately 60% of the eels crossed the cable.
- Westerberg (2000) – Effect of HVDC cables on eel orientation. Technische Eingriffe in Marine Lebensraume. Bundesamt fur International Naturschutzakademie, pp. 1–6. Insel Vlim, Sweden: European eel can detect the B fields emitted by DC cables, however only a small proportion of the eels actively responded to them.
- Orpwood et al. (2015) – Effects of AC magnetic fields (MFs) on swimming activity in European eel Anguilla anguilla: Silver eels were tested in laboratory conditions for their response to an AC magnetic field. 10 eels were tested and no signs of behavioural changes were detected during swimming trials.
- Hvidt et al. (2006) – Impact of high voltage power cable in a Danish OWF on the behaviour of eel was tested; an effect was found however it was unclear whether this was purely due to the electromagnetic field or some other aspect.
3.4.3.2.4 Three-spined stickleback
- No in situ or laboratory experiments identified
3.4.3.2.5 River lamprey
- No in situ or laboratory experiments identified
3.4.3.2.6 Sea lamprey
- No in situ or laboratory experiments identified
3.4.3.2.7 European flounder
- Hvidt et al. (2006) – Impact of high voltage power cable in a Danish OWF on the behaviour of flounder was tested; there was significant effect of the cable, with flounder crossing the cable more often when the electromagnetic field was small than when it was large.
- Bochert & Zettler (2004) – Long‐term exposure of several marine benthic animals to static magnetic fields. Bioelectromagnetics: Journal of the Bioelectromagnetics Society, The Society for Physical Regulation in Biology and Medicine, The European Bioelectromagnetics Association, 25(7), 498-502: Lab study in which juvenile flounder were exposed to a static magnetic field of 3.7 mT for 4 weeks. Only mortality was recorded and no impacts on physiology were tested.
3.4.3.2.8 European smelt/sparling
- No in situ or laboratory experiments identified
3.4.3.2.9 Allis shad
- No in situ or laboratory experiments identified
3.4.3.2.10 Twaite shad
- No in situ or laboratory experiments identified
3.4.3.3 Authors’ assessment on the potential impact
As salmon and sea trout (smolts and adults) swim in the upper water column, they would be unlikely to be physically near any cabling, with the exception of dynamic cables associated with floating offshore wind. Floating wind farms are projected to become more common in the future, however very little is known about how floating developments may potentially impact diadromous fish. The many knowledge gaps around this topic also include the exact design of these structures, however cables will be higher in the water column where diadromous fish are migrating. Therefore, the likelihood of encounter may be higher for floating wind farm designs. Salmonids also undertake deep dives for feeding so these events may take them closer to the seabed and potentially the EMF created by a power cable, increasing the encounter rate which may lead to a potential impact. Additionally, any export cables in coastal areas would have a higher likelihood of encounter as these are areas that salmon and sea trout move through, especially as the water depth decreases near the shore and fish are more likely to be physically closer to the seabed and cables. The Earth’s magnetic field is a cue used for migration so interference with this could be significant if exposure occurs. Salmon will potentially pass a wind farm at least twice during their migration as a post-smolt and adult return migration, however at least during the post-smolt migration, salmon seem to move through coastal areas quickly so the potential overlap with offshore wind farms is likely to be of limited duration. Overlap with potential export cables may be longer, but again, most studies show salmon smolts moving quickly through the coastal zone. As sea trout spend much more time in the coastal environment (compared with Atlantic salmon), their potential overlap spatially and temporally with sub-sea cables could be important.
European eel use the Earth’s magnetic field as a migratory cue so therefore offshore cables can potentially impact this. However, for adults that move quickly during migration, temporal overlap is likely to be fairly short despite them swimming in deep water possibly close to the seabed and power cables. However, in coastal zones the depth use of eels may be more variable and therefore the likelihood of encountering EMFs in shallow coastal areas is higher. Potential impact on juvenile glass eels is difficult to assess, but as an earlier developmental stage, they maybe more sensitive. Furthermore, glass eel swim actively and it has been shown that they have a magnetic compass linked to the tidal cycle, therefore EMFs from cables could possibly have an impact on navigation or there may be physiological effects, but there is currently no evidence for either.
Stickleback seem to swim very close to the surface at sea and therefore they are unlikely to come to close contact with the cables in offshore areas. However, in shallower coastal areas, similar to other species, encounters with EMFs are more likely. It is not known if and how they may sense the electromagnetic fields.
There are very little reported data on the marine depth use of river and sea lampreys and the sensitivity of lampreys to electric and magnetic fields, and therefore it is very difficult to assess potential impacts on these fish.
As flounder is a benthic species, it is likely to come within a few metres of offshore power cables, making it potentially vulnerable. There is evidence of some avoidance behaviour around offshore wind arms and power cables but the overall potential impact is unlikely to be significant, and as a fairly mobile species adults are able to move and avoid the cables if there is an impact
There are very little reported data on the marine depth use of allis and twaite shad and the smelt, and the sensitivity of these species to electric and magnetic fields, and therefore it is very difficult to assess potential impacts. Considering the likely coastal marine distribution of these species, they may be unlikely to come across cables within the wind farms that are often at some distance from the mainland, however in the case of coastal export cables, shad could be impacted. The very limited freshwater distribution of these species will mean that only nearby developments and export cables may potentially cause impacts, however as the full extent of the marine migrations are still unknown it is not possible to assess the likelihood of potential overlap and impact. All conclusions are the authors’ opinions based on extrapolation from available evidence and have been formed with low confidence.
3.4.3.4 Key evidence gaps / recommendations for future research
Description: Despite there being increasing amounts of research on the potential impacts of EMFs, there are still several evidence gaps, and the research focus has been mainly on certain species or groups. However there has been emerging research on other taxa including studies on fish, crustaceans and bivalves. More background on this topic is provided above
To address the identified knowledge gaps, it is recommended that a combination of laboratory and field studies are conducted. Outlined below are a series of sub-questions and the appropriate methodology that would be required to answer each question.
3.4.3.4.1 Characterisation of EMFs
There is a lack of available data on the EMFs emissions from different locations and understanding of temporal-spatial variation in relation to fluctuating power, highlighting a lack of replication. Improved data sharing practices from operating companies as well as collaborations between companies and researchers to gain accurate measurements and validation of models using realistic scenarios of these effects would reduce this uncertainty. This is foundational to understand any potential impacts of EMF generated by offshore wind farms on fishes and put current available studies into context.
3.4.3.4.2 Response of diadromous fish to cable EMFs
The response of diadromous fish to anthropogenic electric and magnetic fields remains largely unknown. The levels at which EMFs (natural and anthropogenic) may be detected or behavioural changes occur is required to understand any potential impact from offshore developments. Study design should account for establishing the levels at which fish can detect natural and anthropogenic EMFs and the levels where there is a response, which may be very different. This information needs to be collected for different life stages as it is likely that the potential effects are different for eggs, juveniles and adults. Focus needs to be both on behavioural and physiological effects.
3.4.3.4.3 Navigation and safe distance for EMF
A key concern with regards to diadromous fish, especially Atlantic salmon, anadromous brown trout and European eel is that the EMFs produced by power cables could be strong enough to cause issues with navigation. To answer this question, the encounter rate (if and how often an individual comes in contact with the EMF) needs to be established. For example, as Atlantic salmon and anadromous brown trout swim in the top few metres of the water column, it is unclear if power cables >20 m below them would be encountered and subsequently have a potential impact. Therefore, the distance at which fish migration/movement may be encountered needs to be established to then understand the potential impact. This requires understanding of the movement ecology of the fish in the vicinity of the cable in addition to the operational characteristics of the cable – as it is the combination of the two that determines the level of EMFs the animal encounters. Future studies need to include traditional and floating turbines to account for the potential differences in emissions and how they target species differently.
When addressing the earlier knowledge gaps, a two-stage approach is proposed. An initial, well-controlled mesocosm laboratory study that replicates the expected cable EMFs would be followed by a series of field studies. It is recommended lab studies include swim trials where fish are exposed to different levels of EMFs. Field studies should include telemetry studies to study responses of individual fish but also methods for investigating group level responses to cables. All studies in the field and lab should include detailed measurements of the EMFs so these can be paired with fish behaviour and physiology. It is important that both laboratory and field studies are replicated for different life stages. Studies should also consider freshwater life stages and habitats as the transfer of power onshore may require cabling to be situated in estuaries and rivers.
General notes
Spatial and temporal scales of data collection: Mesocosm and laboratory studies need to be replicated with a range of EMFs to which fishes may be exposed. In addition, field studies would require replication between sites and over long enough time periods to account for variations in environmental conditions and cable power output.
Feasibility and challenges: For field studies, the main challenge is controlling environmental parameters and ensuring studies have appropriate controls and therefore disentangling any behavioural changes caused by EMFs from, for example, water currents. Furthermore, the best methodology would be using acoustic or satellite telemetry however the probability of the study animals interacting with any power cable is likely to be very low, therefore leading to very small sample sizes despite effort. This could be mitigated by ensuring a large initial number of tagged individuals, transporting study animals closer to the study site or targeting species that are known to be resident in the area. Controlled laboratory/ mesocosm studies may be the best approach to gain an understanding of fishes’ response to EMFs and studying the magneto- and electroreceptive abilities of fish, however these studies can be challenging for assessing natural behaviours (as behaviour in laboratory conditions is never fully natural) and especially if focusing on navigational cues that are best studied in the field. However, well-designed laboratory studies will be able to answer many of the key questions and are likely to strengthen field-based studies when combined.
Species targeted: All but especially the 3 species that are a qualifying interest in the Scottish SACs (Atlantic salmon, river lamprey, sea lamprey).
3.4.4 Novel habitat construction
Construction of offshore wind farms includes building up to hundreds of foundations for the main shaft to support the turbine and the blades. There are several different foundation types used but the most common types are, steel monopile foundation, steel jacket foundation and floating foundation (Hammar et al., 2010). Offshore wind farms are usually located far from the coast in areas that have no existing hard structures in the water column, therefore there is usually a loss of some habitat types (soft sediment) and creation of novel habitat in the form of hard vertical habitat (shafts) and a range of horizontal habitats (foundations) which can thus be considered as artificial reefs or fish aggregating devices (Andersson, 2011; Degraer et al., 2020). The unique aspect of offshore wind turbine foundations in comparison to many other types of artificial reefs is that they cover the whole depth of the water column, and therefore provide a range of habitats from the splash zone to deep subtidal zone (vertical zonation) for different species (Degraer et al., 2020).
Building new offshore structures has the potential to lead to a range of changes including introducing physical barriers, community change, predator-prey interactions, increased disease risk, increased suspended sediment and changes in fishing activity. Many of these potential impacts will also act in conjunction which makes estimating the magnitude of the impact challenging.
3.4.4.1 Community change & predator-prey interactions
Infrastructure associated with a new fixed foundation offshore wind farm will go through a community structure succession (a gradual change in the species composition over time). This was studied by Kerckhof et al. (2019) who conducted a 10-year study at a Belgian wind farm after installation. They found that new foundation structures are initially colonised by fouling organisms (i.e. barnacles, mussels, anemones, amphipods and macroalgae), this happened very rapidly. This pioneer stage lasted ca.2 years. It was followed by a species-rich intermediate stage which was characterised by suspension feeders (3 - 5 years), before reaching a likely species-poor climax stage after 10 years mainly dominated by mussels. This study highlights the importance of long-term studies, as shorter investigations may wrongly conclude that turbine foundations create species-rich ecosystems (Kerckhof et al., 2019). Additionally, there is evidence that communities established on man-made hard structures differ from those that are natural, with species number and Shannon-Wiener Index (a measure of biodiversity in a given ecosystem or community) being lower in the former (Wilhelmsson & Malm, 2008). Although very different, floating structures still have large surface areas for colonisation in the upper water column in the form of multiple mooring chains and dynamic cables rising from seabed.
Some flatfish species appear to respond positively to the new habitat created by offshore wind farms. Buyse et al. (2023) found that plaice (Pleuronectes platessa) around offshore wind farms had higher stomach fullness index and Fulton’s K index than plaice from control areas. Additionally, the wind farm sites had individuals that were larger and with a significantly higher proportion of females.
Novel habitat creation can have positive and negative effects. As described above, there will be an initial increase in species diversity, which may not be a long term (>10 years) effect. This can be followed by the appearance of lower trophic level species and predator species (fish, birds and marine mammals) (Reubens et al., 2014). This has the potential of leading to increased predation risk; however there is also evidence of avoidance for some predator species.
Increases in the number of cod (Gadus morhua) at a wind farm site have been reported in Belgium with telemetry and stomach content analysis showing evidence of crepuscular activity patterns, suggesting intensive feeding at sunset and sunrise (Reubens et al., 2014). Atlantic cod is known to prey on Atlantic salmon smolts (Hvidsten & Lund 1988; Hedger et al., 2011) and may also prey upon the juveniles of the other focal species in this review.
There is evidence of both avoidance and attraction of marine mammals to offshore wind farms. Teilmann & Carstensen (2012) who conducted a 10-year study with a Before-After-Control-Impact (BACI) design at a Danish offshore wind farm found that there was a significant decline in harbour porpoise (Phocoena phocoena) echolocation activity within the wind farm, and even after 10 years it had not recovered to pre-construction levels. There was however evidence of some increase, suggesting that the porpoise population was slowly habituating to the wind farm. Lindeboom et al. (2011) found opposing results in a Dutch offshore wind farm, with an increase in harbour porpoise echolocation activity during an offshore wind farm operational phase. They suggested that this could be due to a reef or shelter effect. In a two-year study in two Danish offshore wind farms, Diederichs et al. (2008) found that harbour porpoises were present inside and outside wind farms almost daily and no differences could be detected between numbers inside and outside the wind farms at either site. While not an offshore wind farm, Todd et al. (2022) investigated the effects of an offshore gas platform construction and operation on harbour porpoises in Dogger Bank in the North Sea. The authors found that, during a 2-year period of initial construction and drilling, there was a significant decline in porpoise detections but 5 months post-construction these returned to baseline levels. Lindeboom et al. (2011) also found that while harbour seals avoided the wind farm during construction, they were found to use it during operation. Many studies suggest that there is an initial decline in porpoise activity during the construction phase of offshore facilities, however in many locations the numbers return to pre-construction rates after some time and in some cases even increase.
Similar results have been found for piscivorous bird species. In a site in the Netherlands, numbers of great cormorant (Phalocrocorax carbo) significantly increased after an offshore wind farm was built, while numbers of common guillemot (Uria aalge) and northern gannets (Morus bassanus) decreased (Leopold et al., 2012). Similar results were found in a Belgian offshore wind farm, common guillemots and northern gannets significantly decreased while lesser black-backed gulls (Larus fuscus) and herring gulls (Larus argentatus) were attracted to the farm (Vanermen et al., 2015). Multi-year studies are required to establish the potential long-term impacts on the ecology of a changing animal community.
As discussed above, man-made structures are usually associated with lower species richness (Wilhelmsson & Malm, 2008). This makes these habitats more vulnerable to invasive species, as there are open niches available for colonisation. Additionally, the locations of most offshore wind farm sites are in areas that usually lack hard substrate, and as such can act as “stepping stones” for the spread of invasive species. Glasby et al. (2007) found artificial structures had higher numbers of non-native species than nearby natural hard substrate in Australia. Page et al. (2006) reported the presence of invasive species at a US offshore oil platform in the Pacific, and Sammarco et al. (2004) noted how oil and gas platforms in the Gulf of Mexico were an important mode for a range of expansion for coral species. Any changes in the community structure and introduction of non-native species may also have consequences for diadromous fish.
3.4.4.2 Increased risk of disease
Disease risk may be higher in offshore wind farm areas due to the artificial reef effect and the higher number of species and individuals coming into contact with each other. One potential pathogen that may be causing an impact is Anisakis simplex (ascaridoid nematode) which causes Red Vent Disease in salmonids. This parasite requires a mammalian host before developing into a sexually mature adult and therefore the potentially increased number of seals and cetaceans at wind farm sites could provide a larger number of potential hosts.
The topic of potential increased pathogen transmission at offshore wind farms has not been investigated and therefore it is not possible to determine if this is a significant risk. However, it is possible that this may be a potential impact to consider.
3.4.4.3 Sediment Disturbance
Construction of offshore wind farms can in certain conditions cause sediment disturbance, impact microbial communities and increase pollution. Wang et al. (2023) investigated how offshore wind turbines affect marine sediment quality and microbial community in Bohai Bay, China. The authors reported that heavy metal concentrations were higher within the wind farm area and the microbial community reflected the sediment quality. Marine sediments can act as sinks for pollutants and contaminants that have entered the environment through human activities, and therefore when sediment is disturbed during foundation construction the contaminants may be reintroduced into the water (Zaborska et al., 2017). The seafloor in POAs may also be a blue carbon sink – an oceanic ecosystem that captures and stores carbon – and physical disturbance could lead to release of long term store carbon (Cunningham & Hunt, 2023).
Increased turbidity at offshore wind farm sites could be due to suspended sediment or detritus produced by the newly established hard substrate epifauna (Baeye & Fettweis, 2015; van Berkel et al., 2020). Vanhellemont and Ruddick (2014) used Landsat-8 imagery to reveal that on two UK wind farms, significant tidal-induced suspended sediment plumes could be observed that were 30-150 m wide and extended more than 1 km downstream from the turbine. Additionally, offshore farm sites may have increased vertical mixing compared to natural habitats (Floeter et al., 2017). Suspended sediment may potentially impact fish in a variety of ways, the severity of potential impact is likely to vary between life stages (eggs, larvae, juveniles, adults) and the effects may be lethal or sub-lethal (Engell-Sørensen & Skyt, 2001). For diadromous fish, increased turbidity could potentially negatively impact feeding opportunities or predator avoidance. Potential impacts are likely to be complex and species-specific, depending on the species’ reliance on visual cues.
One of the main potential impacts of suspended sediment can be damage to eggs and larvae. Out of the 10 focal species in this review, only European flounder breeds in the coastal marine environment. They are a broadcast spawner and therefore their eggs may be at risk from increased turbidity. In turbid conditions, the eggs of pelagic spawners such as cod (Gadus morhua) may be at risk of sinking due to the suspended sediment (Rönbäck & Westerberg, 1996). Auld & Schubel (1978) tested the impacts of different concentrations of suspended sediment on the eggs and larvae of yellow perch, white perch, blueback herring, alewife striped bass and American shad. The authors found that concentrations up to 1000 mg l-1 did not have an impact on the hatching success of eggs of yellow perch, blueback herring, alewife or American shad, however white perch and striped bass eggs were significantly affected. Larvae were more sensitive, and concentrations up to 500 mg l-1 significantly reduced survival for striped bass, yellow perch and American shad. American shad larvae were the most sensitive with concentrations of 100 mg l-1 having a negative effect.
The risk of turbidity to adult fish is mainly due to the sediment coating gill epithelia. Plankton eating fish with long gill rakers (in contrast with carnivorous fish) may be more sensitive to suspended sediment (Engell-Sørensen & Skyt, 2001), as increased turbidity could reduce visibility which may influence prey searching and lead to more particulate material on the gill rakers. Adult fish that are mobile may be able to avoid poor conditions and water quality, assuming that the impacted area is reasonably small. For example, adult salmonids show avoidance of turbidity concentrations above 100 mg/l (Newcombe & MacDonald, 1991).
3.4.4.4 Reduction in fishing activity
Although some of the potential impacts of offshore wind farms are negative, there could also be some positive impacts associated with construction of these developments (Püts et al., 2023). Creation of offshore wind farms may lead to reduced fishing activity in the area, either due to access to the area being limited or voluntary avoidance. However while entering offshore wind farms is banned in some countries (i.e. Belgium), in the UK outside the construction phase, navigation through and trawling in an offshore wind farm is allowed at their own risk. Cessation or reduction in fishing within an area would decrease the risk of fish mortality/removal. There may also be other less direct effects. For example, with regards to bottom trawling, there would be reduced disturbance of benthic habitats and communities. Reduction in fishing activities using bottom-contacting mobile gears is likely to have the greatest positive effect. Dunkley and Solandt (2022) found a 77 % reduction in fishing rate across 12 studied offshore wind farm sites. A review by Halpern (2003) showed that fisheries closures lead to healthier ecosystems with a higher biomass of fish. This effect is likely to only happen within an offshore wind farm however, and therefore only benefits species resident in the area (cf. migratory species). As the ‘artificial reef effect’ increases fish attraction to the structures; there may be an increase in fishing effort around the edges of an offshore wind farm. This has the potential to negatively impact fishes as fishing effort could be concentrated around a relatively small area and fishing activity (trawling) has the potential to lead to bycatch of diadromous species that may be passing the area.
There is evidence of Atlantic salmon, sea trout, European eel, river lamprey, sea lamprey, European flounder, European smelt, allis shad and twaite shad being caught as bycatch in commercial fisheries in the waters around the UK (Elliott et al., 2021; Elliott et al., 2023). Additionally, stickleback have been caught in scientific smolt trawling surveys (Marine Directorate, pers. comm.) However, these numbers are very small and therefore, based on these data, it is unlikely that fisheries in their current form represent a population level risk for these species.
3.4.4.5 Expert panel
Similar to the other potential impacts of offshore wind farms, the expert panel agreed that despite its importance, this topic is understudied and currently there are only small amounts of evidence to draw conclusions on. One issue is that for a lot of the field-based work carried out, the study design does not allow clear distinction between wind farm potential impacts and other environmental impacts. The lack of research is likely due to offshore wind farms being a relatively new phenomenon and additionally, there are many logistical challenges of conducting studies, especially telemetry studies, within offshore developments.
It was highlighted that one key potential impact of novel habitat creation is the artificial reef effect - it is well known that wind farm foundations are particularly attractive for juvenile fish (Degraer et al., 2020; Glarou et al., 2020; APEM, 2022) - which could lead to a potential build-up of larger fish like cod, which could predate on passing diadromous fish. It was noted that one potential predator species that could be attracted to offshore wind farms and be a re-introduction to certain areas of Scottish waters is Atlantic bluefin tuna (Thunnus thynnus). This fish species is known to associate with Fish Aggregating Devices (Lopez et al., 2017). However, whether offshore wind structures would have this effect is unknown. Novel structures may also have fitness effects on the fish that inhabit them; work from Californian protected zones has shown that while density does not change, condition and size of individual fish improves.
Another potential impact that was discussed in detail was changes in fishing pressure in and around the wind farm. While fishing within a fixed foundation wind farm development is allowed in the UK, it is unclear how common this is. Floating wind may present difficulties around access for certain types of fishing activity. There is an incentive to it due to the likely higher numbers of fish present, however it also poses a risk due to the potential collision and entanglement risk with the cables and mooring structures. Fishing on the outskirts of a wind farm might be more likely however as that provides some of the benefits without the risks, and the artificial reef effect often extends some distance from the structure. It is well known that fishers are drawn to the edges of protected marine areas. Fishing pressure may have positive or negative potential impacts on diadromous fish, depending on the species that are targeted, although risk of bycatch is possible for all species. However, while there is evidence of diadromous fish getting caught in commercial trawlers, this is relatively rare. Specifically for salmonids in Scottish waters, the expert opinion was that, although anecdotal, it was very rare for pelagic trawlers to capture salmonids. Marine Directorate used to run a monitoring programme for pelagic fish catches however this was stopped in 2010, while demersal fish monitoring continues occasionally. Although this sort of monitoring data are time consuming to collect, they would be very valuable.
In relation to the potential impact of sediment disturbance, it was noted that it is very dependent on water depth and sediment type, with deep water and gravel substrate having much less of an impact than shallow water with muddy sediment. The overall assessment of this potential impact was that it was likely to be of lesser importance for diadromous fish, however this will be species-specific and need to consider what the main sensory abilities of the species are (vision or sound).
The potential increase in disease transmission due to increased density of potential hosts (especially in relation to A. simplex) was discussed but the experts could not provide any references for this topic.
Recommendations for future studies were discussed and the following questions were highlighted: How do salmon interact with wind farms? Would wind farms create a situation where predators that normally do not use the surface waters, would now be attracted to the top layer of water? What are the migration routes taken by diadromous fish and will they overlap with existing developments? It was noted however that it is difficult to test overlap effects empirically due to the challenge of identifying a study population that is likely to move through a wind farm. As a general note for future studies, it was stated that it is important to develop reproducible methodologies to run across multiple sites and create time series data sets.
3.4.4.6 Species-specific related research
3.4.4.6.1 Atlantic salmon
- No in situ or laboratory experiments identified.
3.4.4.6.2 Brown trout
- No in situ or laboratory experiments identified.
3.4.4.6.3 European eel
- Bergström et al. (2013b) – Study of the Fish Communities at Lillgrund Wind Farm: Final Report from the Monitoring Programme for Fish and Fisheries 2002–2010: 300 eels were acoustically tagged and 100 provided useful information. Similar numbers of eels passed a transect at a wind farm during baseline and operational periods, suggesting that the wind farm did not seem to be a physical barrier that the eels were avoiding. There was however a difference in time taken to move past the wind farm between higher and lower production, with higher production leading to longer migration times. Additionally, the eels were less likely to be detected within the farm at lower production levels.
3.4.4.6.4 Three-spined stickleback
- No in situ or laboratory experiments identified.
3.4.4.6.5 River lamprey
- No in situ or laboratory experiments identified.
3.4.4.6.6 Sea lamprey
- No in situ or laboratory experiments identified.
3.4.4.6.7 European flounder
- No in situ or laboratory experiments identified.
3.4.4.6.8 European smelt/sparling
- No in situ or laboratory experiments identified.
3.4.4.6.9 Allis shad
- No in situ or laboratory experiments identified.
3.4.4.6.10 Twaite shad
- No in situ or laboratory experiments identified.
3.4.4.7 Authors’ assessment on the potential impact
All 10 species of focus for this review, especially during their juvenile life stages, could be negatively affected by changing and increasing predator assemblages, however the lack of studies on this topic limits the interpretations that can be drawn with any confidence. The likelihood and magnitude of this impact will be dependent on the amount of time spent by the species at or around wind farms. Previous work has shown that Atlantic salmon smolts move through coastal areas fairly quickly (9-40 km/day; Lilly et al., 2023) and therefore they may be less likely to be impacted. Similar data are not available for adult salmon and therefore it is not possible to say whether they would be more likely to spend time near offshore wind farms. Similar, directed, long-distance migration is undertaken by the European eel and therefore it is unlikely that there would be a long overlap with coastal areas – however these data do not exist for adult eels in the UK and no data are available for larval stages. Sea trout spend their marine stage (which may last from weeks to months) in the coastal zone resulting in them being the most vulnerable to overlap with offshore developments. Similar to sea trout, European flounder are also commonly found in the coastal zone. No clear movement data are available for three-spined stickleback, sea lamprey, river lamprey, European smelt, allis shad or twaite shad and therefore the temporal and spatial extent of their marine migration is not known.
Increased turbidity due to suspended sediment has the potential impact on all species. Of the 10 focal species in this review, European flounder is the only one that spawns in the coastal marine environment. For the other nine species, this will not be a risk for their eggs. Juveniles of European eel, European flounder, allis shad and twaite shad may be impacted, however, as they migrate or enter marine waters at a young age. The risk to migrating adults is deemed low, however as all species are likely to rely on visual cues for predator avoidance, the potential for reduced visibility near a wind farm may have a potential impact. In terms of feeding, European smelt, which is (mostly) planktivorous, is the likely fish species to be impacted most by suspended sediment.
As there is evidence that of all of the 10 focal fish species within this review are caught in fisheries bycatch, these fish could benefit from a reduced fishing activity. However, on a population level, the potential impact of fisheries bycatch is likely to be very small.
3.4.4.8 Key evidence gaps / recommendations for future research
Description: Currently there is mixed evidence on the potential impacts of offshore wind farms, especially in relation to potentially increased predation risk that is linked to novel habitat creation (artificial reef effect). Some studies have shown an increase in the presence of marine mammals and piscivorous birds whilst others have reported a decrease in their presence in the vicinity of wind farms. It is likely that this is driven to some extent by site-specific variation and is dependent on unique site characteristics, for example the distance of an offshore wind farms from the coastline.
To address the identified knowledge gaps, it is recommended that a combination of laboratory and field studies are conducted. Outlined below are a series of sub-questions and the appropriate methodology that would be required to answer each question.
3.4.4.8.1 Changes to predation risk and changes to prey availability for diadromous fish
To address whether any development may change or increase the predation risk for diadromous fish or change the prey availability for diadromous fish, a detailed understanding of how offshore wind developments can change community structure is required. All changes will have wider ecosystem and food web consequences and therefore an assessment of the whole community is required, but with a particular focus on those species that may predate on or be predated upon by diadromous fish. Furthermore, it is fundamentally important to establish if there is an overlap between the POAs and diadromous fish; an increase in predators will not have an impact on diadromous fish if they are not found near the sites. Therefore, both the risk and likelihood of interaction need to be considered.
A combination of methods should be used to answer these questions. As there is likely to be site-specific variation, the best results will be gained through a BACI study design approach, where sampling is started before construction begins and continues after construction to determine pre- and post-construction impacts. However, a BAG (Before, After, Gradient) design could also provide useful data. (For a comparison of BACI and BAG designs in relation to fisheries surveying at offshore wind farms please see Methratta (2020).) Best results will be gained through combining multiple methods; sea bird counts, trawling surveys, hydroacoustics, baited traps and cameras, eDNA surveys, acoustic monitoring of marine mammals and soundscape surveys. For fish, eDNA may be the best method to begin the survey process by establishing the presence of species in the area and following these results, other methods can be used to quantify the numbers of individuals of different species. It will be then possible to establish whether there is a higher risk of predation and a higher availability of prey species for diadromous fish species.
3.4.4.8.2 Changes to disease risk to diadromous fish
The Red Vent Disease that is found in Atlantic salmon and anadromous sea trout is caused by a parasite called A. simplex which requires a marine mammal host as part of its life cycle. It has been suggested that with the potentially higher number of potential hosts and interactions between different host species, artificial reefs similar to offshore wind farms may be sites for higher disease risk. However as individual turbines are still quite far apart (>1 km), it will be important to establish if increased interaction is likely. This effect is also likely to differ between fixed and floating wind farms that have different mooring systems. Using a combination of the methodologies outlined above a quantification of the presence of marine mammals could be carried out. The marine mammals could act as reservoirs for the disease and transmit it to salmonids.
Another potential source of data, although not providing a direct link, could be using the records of reported cases of red vent disease provided by anglers. This information has been collected by Scottish Government for the last few years and we recommend that this should continue. It would be valuable to see if the number of cases of red vent increases and if there is any association with certain geographical areas. The spatial distribution of this data is very important.
3.4.4.8.2 General notes
Feasibility and challenges:
For best results, these studies require long time frames and there will be considerable spatial variation in potential responses. These studies will take 5-10 years to provide answers as there should be several time points post-construction where studies should be undertaken to account for the habituation of species. However, monitoring shorter time frames (2-3 years; ideally one year pre-construction and one to two years post-construction) will also provide valuable data. Another shorter-term study option is investigating the prevalence of predation within a wind farm with acoustic telemetry (using either sensor tags with temperature and depth or special predation tags), where a spatial gradient could be incorporated into the study design.
By design, these are large-scale, long-term studies which will require considerable time, manpower and funding.
Contact
Email: ScotMER@gov.scot
There is a problem
Thanks for your feedback