Draft Fisheries Assessment – Stanton Bank Reef SAC: Fisheries management measures within Scottish Offshore Marine Protected Areas (MPAs)
These assessments look at the fishing activity occurring within each offshore MPA and SAC and assess the potential impacts of this activity on the protected features within each site. This assessment is for Stanton Bank Reef SAC.
3 Part B Assessment – Fisheries Assessment
3.1 Fisheries assessment overview
Part B of this assessment meets the requirements for an appropriate assessment under Article 6(3) of the Habitats Directive 1992 and Regulation 28 of the Conservation of Offshore Marine Habitats and Species Regulations 2017.
The fishing activities and pressures identified in Part A, at the levels identified in the relevant date range which have been included for assessment in Part B are mobile demersal fishing (trawls, seines, and boat dredges) and static demersal fishing (traps/creels). The only pressures associated with these fishing activities that have been included in Part B are:
- abrasion/disturbance of the substrate on the surface of the seabed;
- penetration and/or disturbance of the substrate below the surface of the seabed, including abrasion;
- changes in suspended solids (water clarity);
- smothering and siltation rate changes (light); and
- removal of non-target species
3.2 Fishing activity descriptions
3.2.1 Existing management within Stanton Banks SAC
No existing fisheries management measures or other fisheries restrictions were identified within the site.
3.2.2 Fishing activity within Stanton Banks SAC
The Stanton Banks SAC overlaps ICES rectangles 41E1 and 41E2 in the West of Scotland (ICES Division 6a), in the Hebrides and Minches and Malin Sea regions. The aggregated gear methods used in Stanton Banks SAC by UK vessels are demersal trawls, pelagic fishing, and traps/creels; demersal seines are used by foreign vessels (Table 3, in Section A). Pelagic fishing (mid-water trawls) was not considered capable of affecting the reef feature of Stanton Banks Reef SAC as there is no contact with the seabed, and so is not considered further. Demersal trawls, demersal seines, and traps/creels are considered in more detail in the following sections.
In addition to UK activity, vessels from Ireland (38 vessels), Norway, France, Netherlands, Germany and Spain (number of vessels cannot be disclosed) may also operate in the site, based on VMS data from 2015-2019. However, it is not possible to accurately determine the gear types associated with the VMS data for these non-UK vessels, or whether they were actively fishing at the time.
3.2.3 Demersal trawls
The aggregated gear method of demersal trawls includes multiple gears that operated within the Stanton Banks SAC between 2015 and 2019. These include bottom otter trawls, multi-rig trawls, and nephrops trawls. Similar pressures are exerted by the different gears used for demersal trawling, subsequently the aggregated gear type of ‘demersal trawl’ was used to map activity across the site.
According to VMS intensity averaged over 2015 to 2019, demersal trawling is concentrated in the northern part of the site (48 – 168, 24 – 48, and 12 – 24 fishing hours per year per grid cell), with a small additional area of higher concentration in the southwest part of the site (12 – 24 fishing hours per year per grid cell). The remainder of the site has lower fishing intensity (less than 12 fishing hours per year per grid cell) with demersal trawling activity absent from the central part of the site (Figure 2).
Swept-Area Ratio (SAR) information averaged over the same time period shows similar patterns of fishing intensity as the VMS data (Figure 2). The highest SAR values are in the northern part of the site (cells swept 2 – 5 or 1 – 2 times per year per grid cell). The rest of the site had low SAR values (cells swept less than once per year per grid cell) with no demersal trawling activity in the central part of the site.
There may be some overlap between the areas exposed to demersal trawl activity and the reef feature.
3.2.4 Demersal seines
The only demersal seine activity within the site between 2015 and 2019 was conducted using foreign vessels, hence specific demersal seine gear type operating within the Stanton Banks SAC is unknown. Fishing with this gear is referred to as the aggregated gear type of ‘demersal seines’ in the following sections to align with the approach taken for the rest of the assessment.
According to VMS intensity averaged over 2015 to 2019, demersal seine activity is very low within the site. The only locations with demersal seine activity (less than 12 fishing hours per year per grid cell) are generally restricted to small areas in the northeastern and southwestern parts of the site (Figure 3).
Swept-Area Ratio (SAR) information averaged over the same time period shows similar patterns of fishing intensity as the VMS data (Figure 3), with low levels of demersal seine activity (cells swept less than once per year per grid cell) in the northeastern and southwestern parts of the site.
There may be some overlap between the areas exposed to low levels of demersal seine activity and the reef feature.
3.2.5 Traps/creels
The only gear within the aggregated traps/creels gear type operating within Solan Banks SAC between 2015 and 2019 was pots/creels. Fishing with this gear is referred to as the aggregated gear type of ‘traps/creels’ in the following sections to align with approach taken for the rest of the assessment.
According to VMS intensity averaged over 2015 to 2019, traps/creels occurred at low levels (less than 12 fishing hours per year per grid cell) with a patchy distribution across the site (Figure 4). Trap fishing was absent from the far northwest and central-north of the site.
Swept-Area Ratio information is not available for static fishing, such as traps/creels. There may be some overlap between the areas exposed to low levels of trap activity and the reef feature.
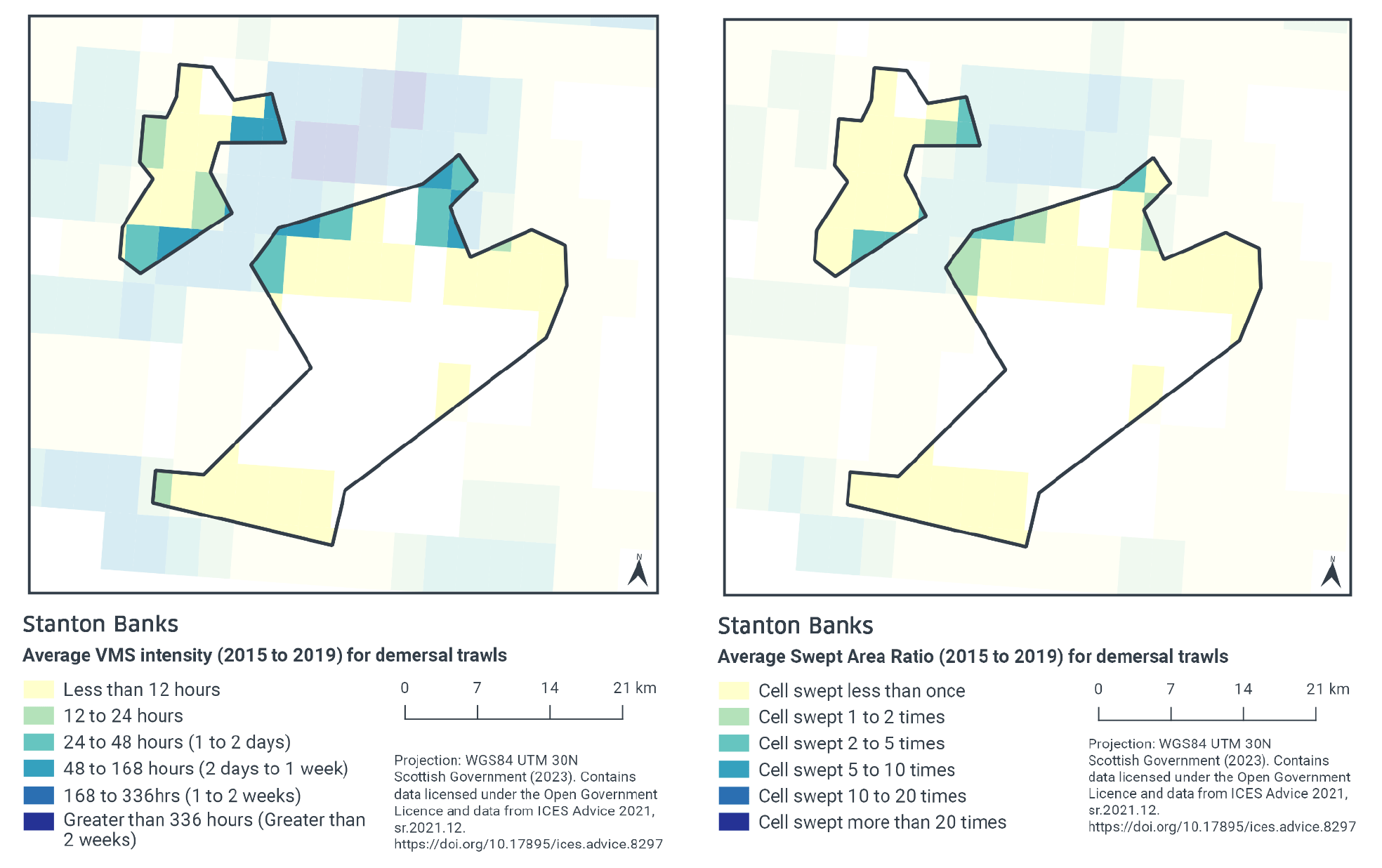
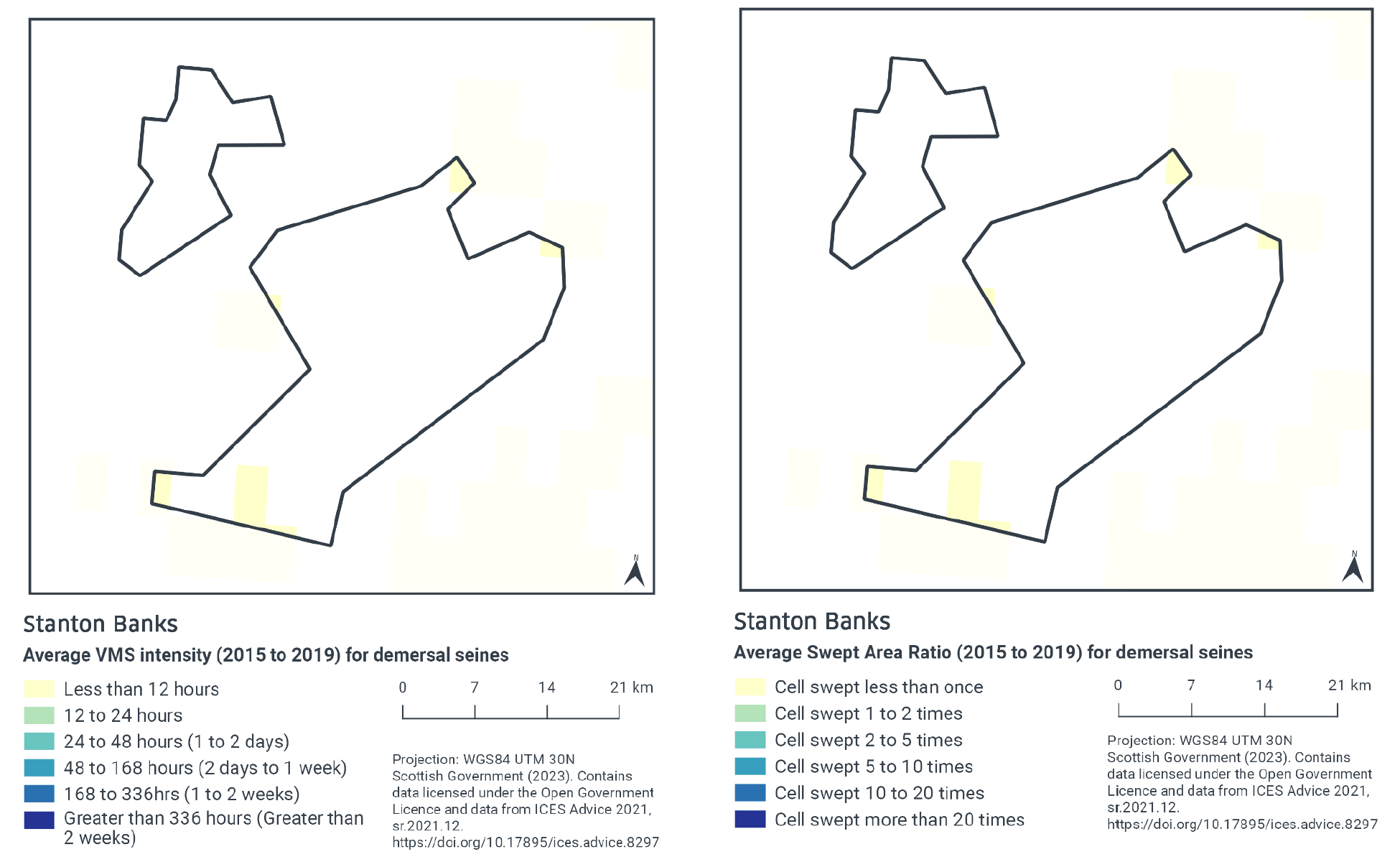
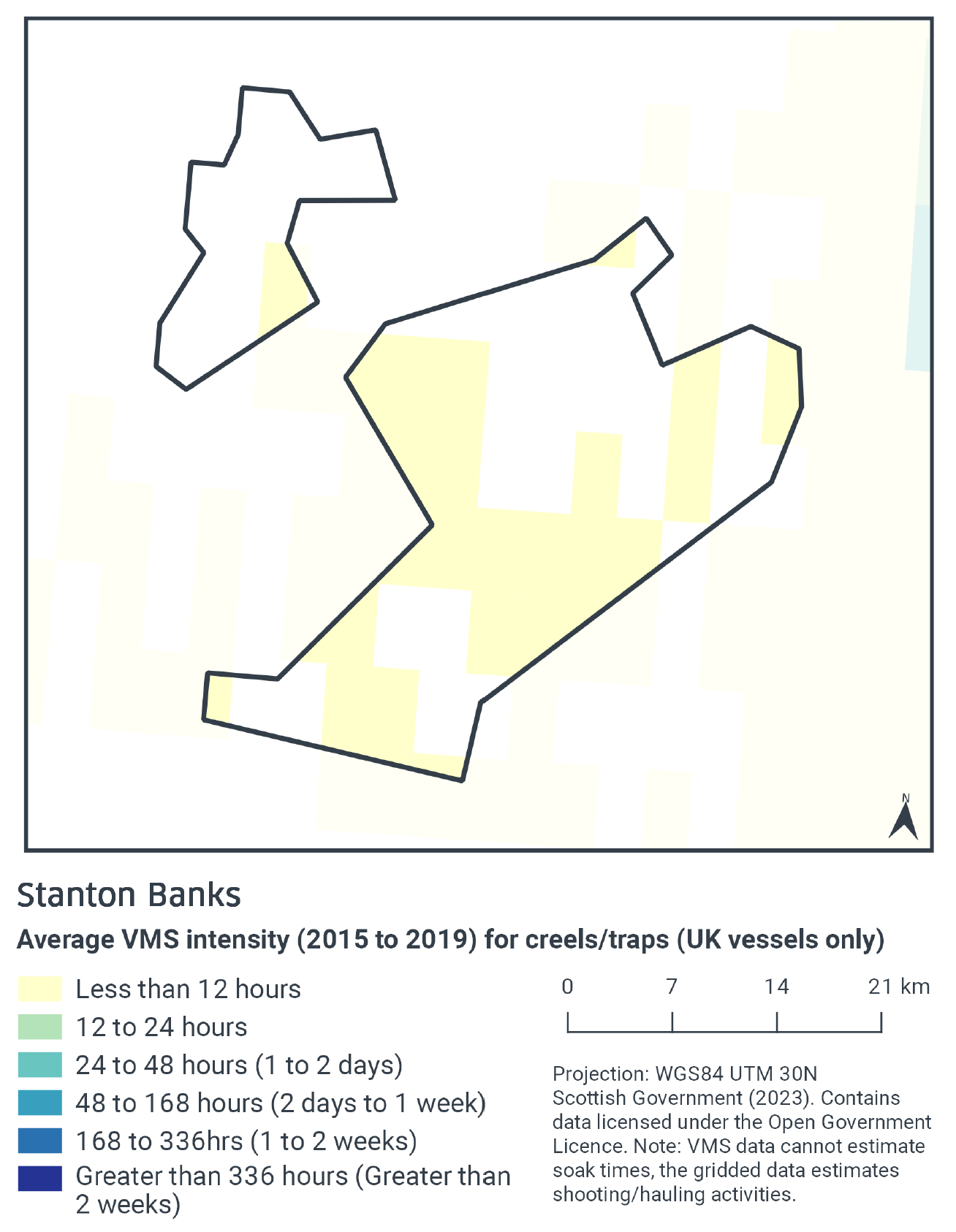
3.2.6 Fishing activity summary
Fishing activities using demersal trawls, demersal seines, and traps/creels all occur within the Stanton Banks SAC. Demersal trawling activity occurs at higher intensity in the northern part of the site, with lower intensity in the central-northern and southern portions, and no demersal fishing in the central section. Demersal seine activity is very low and is restricted to small areas in the north-eastern and south-western parts of the site. Traps/creels have a more patchy distribution across the site and were absent from the far northwest and central-north of the site. Demersal trawls, demersal seines, and traps/creels all have the potential to overlap with the reef feature.
3.3 Fishing activity effects overview
The following sections explore the pressures associated with fishing activity (demersal trawls, demersal seines, and traps/creels) within the Stanton Banks SAC that were identified as potentially having likely significant effects (LSE) on the reef feature. The pressures considered in the following sections are:
- Abrasion/disturbance of the substrate on the surface of the seabed;
- Penetration and/or disturbance of the substrate below the surface of the seabed, including abrasion;
- Changes in suspended solids (water clarity);
- Smothering and siltation rate changes (light); and
- Removal of non-target species.
All five pressures, as exerted by mobile demersal fishing (trawls and seines), were considered to have the potential for LSE. For static demersal fishing (traps/creels), only abrasion/disturbance of the substrate on the surface of the seabed and removal of non-target species were considered to have the potential for LSE.
3.3.1 Abrasion/disturbance of the substrate on the surface of the seabed
According to MarLIN (DeBastos et al., 2023), data on the biotope ‘Brittlestars on faunal and algal encrusted exposed to moderately wave-exposed circalittoral rock’ (CR.MCR.EcCr.FaAIC.Bri) brittlestars are epifaunal and have fragile arms so are likely to be directly exposed and damaged by abrasion. Brittlestars can tolerate considerable damage to arms and even the disk without suffering mortality and are capable of arm and even some disk regeneration (Sköld, 1998). Fishermen tend to avoid brittlestar beds since the animals clog their nets (Jones et al., 2000). However, a passing scallop dredge is likely to remove, displace, or damage brittlestars caught in its path. Although several species of brittlestar were reported to increase in abundance in trawled areas (including Ophiocomina nigra), Bradshaw et al. (2002) noted that the relatively sessile Ophiothrix fragilis decreased in the long-term in areas subject to scallop dredging. Overall, a proportion of the population is likely to be damaged or removed. An average of 36% of individuals in five British brittlestar beds were regenerating arms (Aronson, 1989) which suggests that the beds could persist following exposure to a pressure.
A study by Boulcott & Howell (2011) on the effects of scallop dredging in rocky substrata suggested that associated epifaunal communities, such as bryozoans, hydroids, soft corals and sponges are removed by a passing scallop dredge and that damage to these communities is incremental. The study also recorded that mobile substrata present is likely to be moved and turned as a result of the passing dredge, leading to further damage to the epifaunal communities.
Also according to MarLIN (Stamp et al., 2023) data on the biotope ‘Faunal and algal crusts on exposed to moderately wave-exposed circalittoral rock’ (CR.MCR.EcCr.FaAIC.Bri) this biotope is a subtidal habitat (Connor et al., 2004) and abrasion is most likely to be a result of bottom or pot fishing gear, cable laying etc. which may cause localised mobility of the substrata and mortality of the resident community. The effect would be situation dependent however if bottom fishing gear were towed over a site it may mobilise a high proportion of the rock substrata and cause high mortality in the resident community.
Alcyonium digitatum, Echinus esculentus, Securiflustra securifrons and Spirobranchus triqueter are all sedentary or slow moving species typically associated with the ‘Faunal and algal crusts on exposed to moderately wave-exposed circalittoral rock’ biotope that might be expected to suffer from the effects of dredging. Boulcott & Howell (2011) conducted experimental Newhaven scallop dredging over a circalittoral rock habitat in the sound of Jura, Scotland and recorded the damage to the resident community. The results indicated that the sponge Pachymatisma johnstoni was highly damaged by the experimental trawl. However, only 13% of photographic samples showed visible damage to A. digitatum. Where A. digitatum damage was evident it tended to be small colonies that were ripped off the rock. The authors highlight physical damage to faunal turfs (erect bryozoans and hydroids) was difficult to quantify in the study. However, the faunal turf communities did not show large signs of damage and were only damaged by the scallop dredge teeth which was often limited in extent (approximately 2 cm wide tracts). The authors indicated that species such as A. digitatum and faunal turf communities were not as vulnerable to damage through trawling as sedimentary fauna and whilst damage to circalittoral rock fauna did occur it was of an incremental nature, with the loss of species such as A. digitatum and faunal turf communities increasing with repeated trawls.
Species with fragile tests, such as E. esculentus were reported to suffer badly as a result of scallop or queen scallop dredging (Bradshaw et al., 2000; Hall-Spencer & Moore, 2000). Kaiser et al. (2000) reported that E. esculentus were less abundant in areas subject to high trawling disturbance in the Irish Sea. Jenkins et al. (2001) conducted experimental scallop dredging in the North Irish sea and recorded the damage caused to several conspicuous megafauna species, both when caught as bi-catch and when left on the seabed. The authors predicted 16.4% of E. esculentus were crushed/dead, 29.3% would have >50% spine loss/minor cracks, 1.1% would have <50% spine loss and the remaining 53.3% would be in good condition. Sea urchins can rapidly regenerate spines, e.g. Psammechinus miliaris were found to re-grow all spines within a period of 2 months (Hobson, 1930).
Epifaunal species and communities are considered to be amongst the most vulnerable to bottom gears (Jennings & Kaiser, 1998) and the impact of surface abrasion will depend on the footprint, duration and magnitude of the pressure. Taking all the evidence into account, the MarLIN assessment for the sensitivity of the biotope ‘Brittlestars on faunal and algal encrusted exposed to moderately wave-exposed circalittoral rock’ (CR.MCR.EcCr.FaAlCr.Bri) to abrasion/disturbance of the substrate on the surface of the seabed assessed the biotope as having ‘Low’ resistance, ‘Medium’ resilience, and ‘Medium sensitivity to this pressure. Resistance and resilience will be lower (and hence sensitivity greater) to repeated abrasion events (DeBastos et al., 2023).
The MarLIN assessment for the sensitivity of the biotope ‘Faunal and algal crusts on exposed to moderately wave-exposed circalittoral rock’ (CR.MCR.EcCR.FaAlCr) to abrasion/disturbance of the substrate of the seabed assessed the biotope as having ‘Medium’ resistance, ‘High’ resilience, and ‘Low’ sensitivity to this pressure (Stamp et al., 2023).
3.3.2 Penetration and/or disturbance of the substrate below the surface of the seabed, including abrasion
According to MarLIN, the species characterizing these biotopes are epifaunal, occurring on rock, which is resistant to sub-surface penetration. The assessment for abrasion at the surface only is therefore considered to equally represent sensitivity to this pressure.
The MarLIN assessment for the sensitivity of the biotopes ‘Brittlestars on faunal and algal encrusted exposed to moderately wave-exposed circalittoral rock’ (CR.MCR.EcCr.FaAlCr.Bri) and ‘Faunal and algal crusts on exposed to moderately wave-exposed circalittoral rock’ (CR.MCR.EcCR.FaAlCr) to penetration and/or disturbance of the substrate below the surface of the seabed, including abrasion assessed the pressure 'Not Relevant' for these biotopes (DeBastos et al., 2023; Stamp et al., 2023).
3.3.3 Changes in suspended solids (water quality)
MarLIN (DeBastos et al., 2023) data on circalittoral rock habitats, such as the biotope ‘Brittlestars on faunal and algal encrusted exposed to moderately wave-exposed circalittoral rock’ (CR.MCR.EcCr.FaAIC.Bri), shows these environments are characterized by animal dominated communities (Connor et al., 2004). The brittlestars Ophiothrix fragilis, Ophiocomina nigra and Ophiura albida are passive suspension feeders and a significant supply of suspended organic material is needed to meet the energetic costs of the great numbers of individuals in a brittlestar bed. Brittlestar beds occur in a variety of water flow regimes from sea lochs to more energetic coastal sites (Connor et al., 2004) so are likely to tolerate a variety of different suspended sediment concentrations. For example, dense brittlestar beds occur in the Dover Straits, where the concentration of suspended particles in the water column changes between 18-32 mg l-1 annually (Davoult & Gounin, 1995). Although some brittlestar species are able to perceive differences in light and dark, visual perception is limited (Tillin & Tyler-Walters, 2014) and brittlestars are unlikely to be directly affected by changes in light resulting from a change in turbidity and suspended solids.
However, local increases in turbidity in waters previously within the photic zone may alter local abundances of phytoplankton and surface diatoms, and the zooplankton and other small invertebrates that feed on them. Davoult & Gounin (1995) found that the growth rate of O. fragilis in the Dover Strait was maximal in April/May, coinciding with the spring phytoplankton bloom, which suggests that an increase in suspended solids and resulting increase in turbidity may indirectly reduce feeding efficiency in brittlestars. Nonetheless, since phytoplankton may arrive from distant sources and brittlestars may also feed on organic detritus, any effects are expected to be small. Additionally, O. fragilis has a low respiration rate and can tolerate considerable loss of body mass during reproductive periods (Davoult et al., 1990) suggesting that this species may tolerate feeding restrictions.
Other species occurring in the biotope CR.MCR.EcCr.CarSp.Bri, such as hydroids, bryozoans, Parasmittina trispinosa and Caryophyllia (Caryophyllia) smithii, are suspension feeders and may benefit from an increase in suspended organic matter to some extent. Corals such as C. smithii have cilia on their tentacles capable of clearing silt (K. Hiscock, pers. obs.; cited in Hiscock, 2000) and Hiscock and Howlett (1976) found higher densities of C. smithii in turbid waters which limited the growth of kelps.
Coralline algae, especially the crustose forms that are relevant for the biotope CR.MCR.EcCr.CarSp.Bri, are thought to be resistant to sediment scour (Littler & Kauker, 1984), so any sediment scour resulting from an increase in suspended sediments will probably not adversely affect the coralline crusts at the benchmark level. A moderate change in light resulting from a turbidity change is also unlikely to negatively affect coralline crusts as these are often found in areas with reduced light penetration (Dethier, 1994).
Also according to MarLIN (Stamp et al., 2023) data on the biotope ‘Faunal and algal crusts on exposed to moderately wave-exposed circalittoral rock’ (CR.MCR.EcCr.FaAlCr) A. digitatum, Securiflustra securifrons and S. triqueter are not thought highly susceptible to changes in water clarity due to the fact they are suspension feeding organisms and are not directly dependent on sunlight for nutrition. Alcyonium digitatum has been shown to be tolerant of high levels of suspended sediment. Hill et al. (1997) demonstrated that A. digitatum sloughed off settled particles with a large amount of mucous. Alcyonium digitatum is also known to inhabit the entrances to sea lochs (Budd, 2008) or the entrances to estuaries (Braber & Borghouts, 1977) where water clarity is likely to be highly variable.
Moore (1977) suggested that E. esculentus was unaffected by turbid conditions and is an important grazer of red macro-algae within CR.MCR.EcCr. Increased turbidity and resultant reduced light penetration is likely to negatively affect algal growth. However, E. esculentus can feed on alternative prey, detritus or dissolved organic material (Lawrence, 1975; Comely & Ansell, 1988).
Increased turbidity will reduce light penetration and hence phytoplankton productivity. Small phytoplankton are probably an important food source for filter feeders in the shallow subtidal, such as bryozoans. Although, Flustra foliacea for example is also found at greater depths, where organic particulates (detritus) are probably more important.
According to Bacescu (1972), sabellids are accustomed to turbidity and silt. S. triqueter has also recently been recorded by De Kluijver (1993) from Scotland in the aphotic zone, indicating that the species would not be sensitive to an increase in turbidity.
The evidence presented suggests that an increase in suspended organic matter may be beneficial to some extent by providing increased food material, while a decrease in suspended sediment may reduce food supplies to brittlestar beds. Additionally, increases in suspended solids that involve increase of inorganic particles may interfere with the feeding of brittlestars (Aronson, 1992 cited in Hughes, 1998), particularly in non-current swept areas. However, the biotope occurs in a wide range of conditions and is likely to be adapted to respond to changes in suspended solids at the pressure benchmark and the overall species richness in the biotope is not likely to change.
Taking all the evidence into account, the MarLIN assessment for the sensitivity of the biotopes ‘Brittlestars on faunal and algal encrusted exposed to moderately wave-exposed circalittoral rock’ (CR.MCR.EcCr.FaAlCr.Bri) and ‘Faunal and algal crusts on exposed to moderately wave-exposed circalittoral rock’ (CR.MCR.EcCR.FaAlCr) to changes in suspended solids (water quality) assessed the biotopes as having ‘High’ resistance, ‘High’ resilience, and ‘Not sensitive’ to this pressure (DeBastos et al., 2023; Stamp et al., 2023).
3.3.4 Smothering and siltation rate changes (Light)
According to the MarLIN assessment for the biotope ‘Brittlestars on faunal and algal encrusted exposed to moderately wave-exposed circalittoral rock’ (CR.MCR.EcCr.FaAIC.Bri) (DeBastos et al., 2023), increased siltation on rock biotopes is likely to lead to a decrease in diversity. Material in suspension can affect the efficiency of filter and suspension feeding (Sherk & Cronin, 1970; Morton, 1976). Effects can include abrasion and clogging of gills, impaired respiration, clogging of filter mechanisms, and reduced feeding and pumping rates. Dense beds of brittlestars tend not to persist in areas of excessive sedimentation, because high levels of sediment foul the brittlestars feeding apparatus (tube feet and arm spines), and ultimately suffocates them (Schäfer, 1962 cited in Aronson, 1992). Aronson (1989) referred to the demise of Warner's (1971) O. fragilis bed in Torbay, and tentatively suggested it was due to increased sedimentation caused by the localised dumping of construction materials (Aronson, 1989).
Species such as the soft coral A. digitatum that project above the substratum may not be completely covered with sediment but feeding structures may become clogged. Smaller colonies may be smothered, impairing respiration, and hence adversely affected. Encrusting species (e.g. encrusting sponges, and bryozoans such as Parasmittina trispinosa) are more likely to be completely covered and will probably die (Jackson & Hiscock, 2008). However encrusting corallines and encrusting bryozoans have been reported to survive being overgrown by other species (Gordon, 1972; Sebens, 1985; Todd & Turner, 1988; cited in Tyler-Walters, 2002).
Caryophyllia (Caryophyllia) smithii is small and sessile and would therefore likely be unable to avoid being inundated in a ‘light’ sedimentation event. Although, Bell & Turner (2000) reported C. smithii was abundant at sites of ‘moderate’ sedimentation (7 mm ± 0.5 mm) in Lough Hyne, a light deposition of 5 cm of sediment is likely to adversely affect this cup coral.
In areas of high water flow dispersion of fine sediments may be rapid and this could mitigate the magnitude of this pressure by reducing the time exposed. This would likely be the case in the medium energy biotope CR.MCR.EcCr.FaAlCr.Bri, where ‘light’ deposition of sediments is likely to be cleared in a few tidal cycles. In exposed situations suspended material can cause scour, but this is normally a result of the temporary resuspension of relatively coarse bottom material rather than of fine material in long-term suspension.
Also according to MarLIN (Stamp et al. 2023) data on the biotope ‘Faunal and algal crusts on exposed to moderately wave-exposed circalittoral rock’ (CR.MCR.EcCr.FaAlCr) A. digitatum, S. securifrons and S. triqueter in this environment are sessile and thus, would be unable to avoid the deposition of a smothering layer of sediment. Some A. digitatum colonies can attain a height of up to 20 cm (Edwards, 2008), S. securifrons colonies can attain a height of 10 cm (Porter, 2012) so would still be able to feed in the event of sediment deposition. However, S. triqueter are an encrusting species and would thus likely be smothered, and depending on sediment retention could block larval settlement.
Holme & Wilson (1985) examined the bottom fauna in a tide-swept region of the central English Channel. Flustra foliacea dominated communities were reported to form in areas subject to sediment transport (mainly sand) and periodic, temporary, submergence by thin layers of sand (< 5 cm).
Comely & Ansell (1988) recorded large E. esculentus from kelp beds on the west coast of Scotland in which the substratum was seasonally covered with "high levels" of silt. This suggests that E. esculentus is unlikely to be killed by smothering, however, smaller specimens and juveniles may be less resistant. A layer of sediment may interfere with larval settlement. If retained within the host biotope for extended periods a layer of 5 cm of sediment may negatively affect successive recruitment events. However, high tidal energy within the CR.MCR.EcCr.FaAlCr biotope is likely to remove 5 cm of deposited sediment within a few tidal cycles.
Smothering and siltation rates (light) is not considered to alter the physical reef habitat but there may be effects on the biological community. The key characterizing species of brittlestars are likely to be affected by a single discrete event of light deposition of fine materials that is not removed in the short-term by water movement. Taking all the evidence into account, the MarLIN assessment for the sensitivity of the biotope ‘Brittlestars on faunal and algal encrusted exposed to moderately wave-exposed circalittoral rock’ (CR.MCR.EcCr.FaAlCr.Bri) to smothering and siltation rate changes (light) assessed the biotope as having ‘Low’ resistance, ‘Medium’ resilience, and ‘Medium’ sensitivity to this pressure (DeBastos et al. 2023).
The MarLIN assessment for the sensitivity of the biotope ‘Faunal and algal crusts on exposed to moderately wave-exposed circalittoral rock’ (CR.MCR.EcCR.FaAlCr) to smothering and siltation rate changes (light) assessed the biotope as having ‘High’ resistance, ‘High’ resilience, and ‘Not sensitive’ to this pressure (Stamp et al. 2023).
3.3.5 Removal of non-target species
According to the MarLIN assessment for the biotope ‘Brittlestars on faunal and algal encrusted exposed to moderately wave-exposed circalittoral rock’ (CR.MCR.EcCr.FaAIC.Bri) (DeBastos et al. 2023), fisheries tend to avoid brittlestar beds since the animals clog nets (Jones et al., 2000). However, brittlestars may be damaged or directly removed by static or mobile gears that are targeting other species. Direct, physical removal is assessed under abrasion and penetration pressures. The sensitivity assessment for this pressure considers any biological and ecological effects resulting from the removal of non-target species.
Although several species of brittlestar (including O. nigra) were reported to have increased in abundance in trawled areas, Bradshaw et al. (2002) noted that the relatively sessile O. fragilis decreased in the long-term in areas subject to scallop dredging. The bed may contract in size as individual brittlestars move to re-establish contact with neighbours or the number of low density patches could increase. If water currents were very strong some animals may be washed away as the support provided by other individuals in dense aggregations decreases. In addition, commercial fisheries may discard damaged or dead non-target species. This could result in increased available food supply to scavenging brittlestars but may also attract mobile predators and scavengers including fish and crustaceans to habitats supporting brittlestars, which may alter predation rates.
The remaining defining species in these biotopes, including coralline crusts, C. smithii and P. trispinosa, are sessile and also likely to be incidentally removed as part of an extraction or fishing event.
Also according to MarLIN (Stamp et al. 2023), data on the biotope ‘Faunal and algal crusts on exposed to moderately wave-exposed circalittoral rock’ (CR.MCR.EcCr.FaAlCr), A. digitatum and faunal turf communities (which include bryozoans such as S. securifrons) are probably resistant to abrasion through bottom fishing (see abrasion pressure). Alcyonium digitatum goes through an annual cycle: from February to July all A. digitatum colonies are feeding, and from July to November an increasing number of colonies stop feeding. During this period, a large number of polyps can retract and a variety of filamentous algae, hydroids and amphipods can colonize the surface of colonies epiphytically. From December to February, the epiphytic community is however sloughed off (Hartnoll, 1975). If A. digitatum were removed the epiphytic species would likely colonize rock surfaces and are therefore not dependent on A. digitatum.
Within this biotope, A. digitatum, S. securifrons and S. triqueter spatially compete, however there isn’t any evidence to suggest other interspecific relationships or dependencies between these species. Therefore, removal of one or a number of these species would provide colonization space and most likely benefit the species with rapid colonization rates (e.g. S. triqueter). Echinus esculentus is an important red algae grazer within this biotope (Connor et al., 2004), without which the abundance of red algae may increase and possibly displace some of the faunal turf species. If A. digitatum, S. securifrons and S. triqueter were removed this would alter the character of the biotope.
Taking all the evidence into account, the MarLIN assessment for the sensitivity of the biotopes Brittlestars on faunal and algal encrusted exposed to moderately wave-exposed circalittoral rock (CR.MCR.EcCr.FaAlCr.Bri) and Faunal and algal crusts on exposed to moderately wave-exposed circalittoral rock (CR.MCR.EcCR.FaAlCr) to removal of non-target species assessed the biotope as having ‘Low’ resistance, ‘Medium’ resilience, and ‘Medium’ sensitivity to this pressure (DeBastos et al., 2023; Stamp et al., 2023).
3.3.6 Impacts of mobile demersal gear (trawls and seines)
As detailed in the JNCC Advice on Operations for Stanton Banks SAC, abrasion/disturbance of the substrate on the surface of the seabed and penetration and/or disturbance of the substrate below the surface of the seabed, including abrasion, occur where gear makes contact with the seafloor. The area affected is determined by the footprint of the gear and the amount of movement across the seabed. The different gear components will make variable contributions to the total physical disturbance of the seabed and its associated biota, and hence the pressure will vary according to factors such as gear type, design/modifications, size and weight, method of operation (including towing speed) and habitat characteristics (e.g. topography) (Lart, 2012; Polet & Depestele, 2010; Suuronen et al., 2012). Towed bottom fishing gears are used to catch species that live in, on or in association with the seabed and therefore are designed to remain in close contact with the seabed. That interaction with the seabed can lead to disturbance of the upper layers of the seabed, direct removal, damage, displacement or death of the benthic flora and fauna; short-term attraction of scavengers; and the alteration of habitat structure.
Benthic seines are generally of lighter construction as there are no trawl doors or warps, resulting in less disturbance of the seabed than trawling Polet & Depestele, 2010; Donaldson et al., 2010; Suuronen et al., 2012). As a relative comparison of gear types, otter trawls tend to have less physical impact on the seafloor than beam trawls (and dredges) with their heavy tickler chains, although the doors of an otter trawl do create recognisable scour of the seabed (Hinz et al., 2012; Polet & Depestele, 2010; Lart, 2012; Paschen et al. 2012). The magnitude of the immediate response to fishing disturbance, cumulative effects and recovery times varies significantly according to factors such as the type of fishing gear and fishing intensity, the habitat and sediment type, and levels of natural disturbance and among different taxa (Collie et al., 2000; Boulcott et al., 2014; Kaiser et al., 2006; Hinz et al., 2009; Kaiser et al., 2001).
Changes in suspended solids (water quality) and siltation rate changes (light), including smothering, may result from physical disturbance of the sediment, along with hydrodynamic action caused by the passage of towed gear, leading to entrainment and suspension of the substrate behind and around the gear components and subsequent siltation (Sewell et al., 2007; Gubbay & Knapman, 1999; Lart, 2012; Kaiser et al., 2002; Riemann & Hoffmann, 1991; O’Neill et al., 2008; Dale et al., 2011; O’Neill & Summerbell, 2011). The quantity of suspended material, its spatial and temporal persistence and subsequent patterns of deposition will depend on factors associated with the gear (such as type/design, weight, towing speed), sediment (particle size, composition, compactness), the intensity of the activity, and the background hydrographic conditions (Sewell et al., 2007; Connor et al., 2004; Dale et al., 2011; O’Neill et al., 2008). Turbid plumes can reduce light levels and smother feeding and respiratory organs. Sediment remobilisation and deposition can affect the settlement, feeding, and survival of biota through smothering of feeding and respiratory organs. Prolonged exposure of an area to these pressures may result in changes in sediment composition, including through suspension and transport of finer material. There are also concerns over resuspension of phytoplankton cysts and copepod eggs (Kaiser et al., 2001; Sewell et al., 2007; Gubbay & Knapman, 1999; Kaiser et al., 2002; O’Neill & Summerbell, 2011).
Bycatch (i.e. discarded catch) is associated with almost all fishing activities and is related to factors such as gear type and its design (i.e. its selectivity), the target species and effort. There are significant concerns over the impacts of discards on marine ecosystems including changes in population abundance and demographics of affected species and altered species assemblages and food web structures (Alverson et al., 1994; Kaiser et al, 2001). As with other benthic towed gears, discarding of fish species from demersal seine net fisheries can be significant (Polet & Depestele, 2010; ICES, 2011). These are relatively few studies of the non-fish bycatch composition for demersal seines, however, it is probably similar to that of demersal trawls e.g. crustaceans and other invertebrates, etc., although quantities of such bycatch are likely to be lower than that of other gear types such as beam trawls (Suuronen et al., 2012; ICES, 2011; Donaldson et al., 2010; Walsh & Winger, 2011). Mixed-species and shrimp/prawn demersal trawl fisheries are associated with the highest rates of discarding and pose the most complex problems to resolve (Alverson et al., 1994; Feekings et al., 2012; Catchpole et al., 2005). Benthic trawls most frequently result in bycatch of fish crustaceans and other invertebrates and less frequently turtles and birds (Gubbay & Knapman, 1999; Sewell & Hiscock, 2005; ICES, 2013; Pierpoint, 2000; Bergmann & Moore, 2001; Catchpole et al., 2005; Tulp et al., 2005).
The two biotopes identified from Stanton Banks SAC were not considered to be sensitive to suspended solids (water quality) but were considered to have medium sensitivity to removal of non-target species and low-medium sensitivity to abrasion/disturbance of the substrate on the surface of the seabed.
There is a risk that abrasion/disturbance of the substrate on the surface of the seabed caused by mobile demersal gear (trawls and seines) may not help the achievement of favourable condition. Even if the impacts across gear types vary, mobile demersal fishing gears are likely to have negative impacts on biological communities across the Stanton Banks Reef SAC and these gears are not considered compatible with achieving favourable condition for the Annex I reef feature.
Considering the current levels of mobile demersal trawl and seine fishing activity within the site, and information on the impacts of the pressures associated with these activities, demersal trawling and demersal seining are causes for concern for the reef feature of Stanton Banks SAC. This aligns with the JNCC Conservation Advice Statements (2020), which advise that demersal fishing activity should be managed to restore the Annex I Reefs by reducing or removing the associated pressures.
Through physical impacts from gear interacting with the seabed, mobile demersal gear has the potential to hinder achieving favourable condition for the reef feature. Thus ensuring site integrity in the long term through maintaining or restoring the extent and distribution and structure and function of the qualifying habitat in the site and the supporting processes on which the qualifying habitat relies is required. Accordingly, Scottish Ministers conclude that demersal trawls and seines alone are not compatible with the conservation objectives of the site and may result in an adverse effect on site integrity.
3.3.7 Impacts of static demersal gear (traps/creels)
As detailed in the JNCC Pressures Associations Database, abrasion/disturbance of the substrate on the surface of the seabed can result from surface disturbance caused by contact between the traps/creels along with any associated ground ropes or anchors and the seabed. This occurs during setting of the pots/traps and/or by movement of the gear over the seabed, for example during rough weather or during retrieval. Such physical disturbance can result in epifauna, especially emergent species such as erect sponges and coral, being dislodged (including snagged on the pot) or damaged, although there are limited studies of such effects (Lart, 2012; Polet & Depestele, 2010; Walmsley et al., 2015; Gubbay & Knapman, 1999; Sewell & Hiscock, 2005; Coleman et al., 2013). The individual impact of a single fishing operation may be slight but cumulative damage may be significant (Eno et al., 2001; Foden et al., 2010). It was recently suggested that pot fishing at lower pot densities did not have negative impacts on seafloor communities, although negative effects did occur at higher pot densities (e.g. where pot densities exceeded 15 – 25 pots per 0.25 km2: Rees et al. (2021).
Bycatch (i.e. discarded catch) is associated with almost all fishing activities and is related to factors such as the gear type and its design (i.e. its selectivity), the targeted species and effort. There are significant concerns over the impacts of discards on marine ecosystems, including changes in population abundance and demographics of affected species and altered species assemblages and food web structures (Alverson et al., 1994; Kaiser et al., 2001). Whilst generally considered one of the most selective gear types, pots/traps/creels are associated with bycatch, including of non-target crustaceans (berried females of target species are also considered bycatch in some fisheries, for example), fish, mammals (e.g. seals in cod pots) and potentially some bird species (ICES, 2013; Sewell & Hiscock, 2005; Königson et al., 2015). Bycatch survival rates are generally higher for pots than other fishing gear types (Suuronen et al., 2012; Seafish, 2014). However, the associated ropes can also result in entanglement of turtles and mammals (Sewell and Hiscock, 2005; Pierpoint, 2000). Salmon nets and fyke nets have been associated with bycatch of birds and mammals (Murray et al., 1994; Cullen & McCarthy, 2002; ICES, 2013; Lunneryd et al., 2005), as well as non-target fish species.
Considering the current levels of static demersal trap fishing within the site, and information on the impacts of abrasion/disturbance of the substrate on the surface of the seabed and removal on non-target species, trap fishing is not currently a cause of concern for the reef feature of Stanton Banks SAC.
Given the evidence above, the impacts of abrasion/disturbance of the substrate on the surface of the seabed and removal of non-target species from demersal static gear (traps/creels) alone within Stanton Banks SAC at current activity levels would not hinder achieving favourable condition for the reef feature. Accordingly, Scottish Ministers conclude that demersal static gear (traps/creels) alone are compatible with the conservation objectives of the site at current activity levels and will not result in an adverse effect on site integrity.
3.4 Part B Conclusion
The assessment of fishing pressures at current activity levels on reef features of the Stanton Banks SAC has indicated that an adverse effect on site integrity cannot be ruled out where mobile demersal fishing (demersal trawl and demersal seine) activities occur. As such Scottish Ministers conclude that management measures to restrict mobile demersal gears would be required within Stanton Banks SAC to ensure the integrity of the site. Section 5 contains further details on these potential measures.
Scottish Ministers conclude that the remaining static demersal fishing activities (traps/creels), when considered in isolation and at current levels, are compatible with the conservation objectives of the site and will not result in an adverse effect on site integrity for Stanton Banks SAC.
Contact
Email: marine_biodiversity@gov.scot
There is a problem
Thanks for your feedback