Offshore Wind Sustained Observation Programme (OW-SOP): scoping report
Physical processes are important as they influence the productivity of the phytoplankton which form the base of the entire North Sea ecosystem. This project recommends approaches to assess the potential impact of offshore wind farms on physical processes.
Appendix B. Supporting material (chapter 2)
i. Prevailing physical properties of the North Sea waters
Water circulation
The North Sea is characterized by its broad connection to the ocean and by strong continental impacts from north-western Europe. This results in the substantial interplay of oceanic influences such as tides, the North Atlantic Oscillation (NAO), and the North Atlantic low-pressure systems, as well as continental influences such as freshwater discharge, heat flow, and input of pollutants. These complex interactions generate specific physical and biogeochemical regimes (Sündermann & Pohlmann, 2011).
In the northern half of the North Sea water properties are largely dominated by North Atlantic inflow (Queste et al., 2016). To the south, a small inflow via the English Channel occurs (Figure 2.1). Atlantic waters enter the northern North Sea between Orkney and Shetland and then generally circulate anticlockwise, following the Scottish coast southward before turning east and crossing North Sea north of Dogger Bank (Figure 2.1). Along the west coast of Scotland, the influence of the land run-off from the estuaries and sea lochs significantly reduces the salinity close to the land and impacts the circulation locally.
Tidal currents
North Sea tides are characterized by strong semi-diurnal component, with current speeds ranging from 1.6 cm/s in the Skagerrak to 67 cm/s in the Fair Isle Channel between Orkney and Shetland (Vindenes et al., 2018). There are several localised areas around the Scottish seas that also experience very strong tidal currents (the Pentland Firth, off the Mull of Kintyre and Hebrides).
Otto et al. (1990) provided a map of the rate of tidal energy dissipation for the tide; this is a measure of tidal heating or tidal working which occurs due to tidal friction processes. The results of (Otto et al., 1990) indicate that the southern, more shallow North Sea has higher rates of tidal energy dissipation than the northern North Sea concurrent with the shallower bathymetry there.
The North Sea (and surrounding) waters have been under investigation for decades. Large datasets and monitoring programmes were initiated also due to the O&G industry and its activities in the North Sea waters, providing a legacy of information that can be utilised, if available, to understand how environmental parameters have changed through time. Data collection has been undertaken by academic surveys, statutory authorities and industry; however, programmes and approaches have been driven by different aims and objectives. Below, we highlight some of the relevant data collection/sea-trial programmes completed and underway within the context of OW.
ii. Relevant data collection from past and current programmes
Relevant Scientific projects in the North Sea
The UK project AlterECO (an Alternative framework to assess the marine ECOsystem) took part in the North Sea between 2017 – 2019 with the aim to demonstrate the suitability of solely using Autonomous Underwater Vehicles (AUVs) for monitoring ecosystem health and functioning (Palmer et al., 2020). As part of this project, 19 gliders were deployed providing measurements of temperature, salinity, chlorophyll, turbulence, dissolved oxygen, primary production, nitrate, phosphate and turbidity. The project maintained a continuous 100 km transect over an area in the North Sea prone to seasonal oxygen depletion for 18 months. The Marine Autonomous Robotic Systems (MARS) centre at the National Oceanography Centre (Southampton, UK) was responsible for deploying, piloting and recovering many of the gliders, with a user interface so that scientists were able to monitor the data in real-time and alter missions accordingly (Figure B. 1).
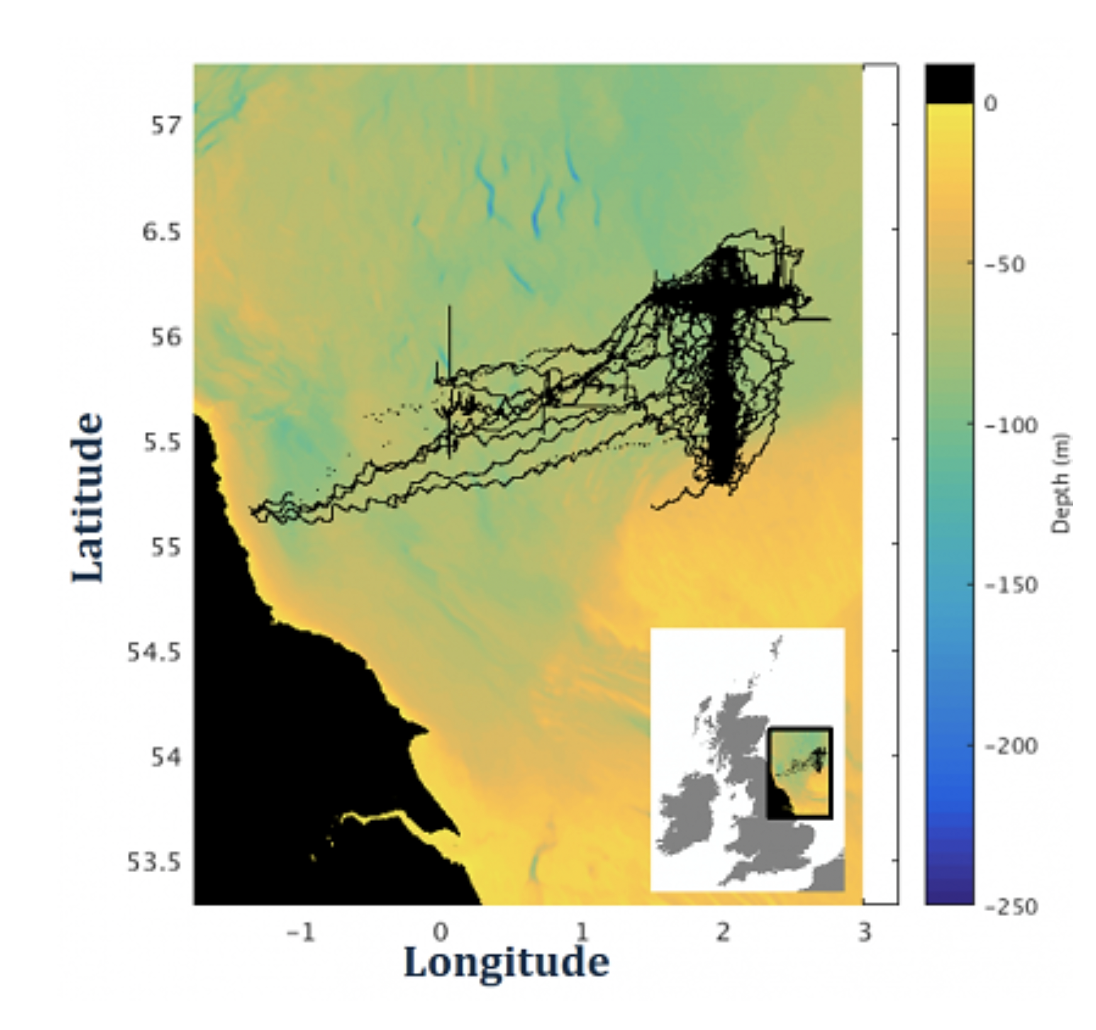
The Marine Autonomous Systems in Support of Marine Observations (MASSMO) project is a pioneering multi-partner series of trials and demonstrator missions that aim to explore the UK seas using a fleet of marine robots. So far, the project is in its 4th phase and has monitored both the marine environment (MSFD Descriptor 7, D7) marine life (MSFD Descriptor 1, D1; Descriptor 4, D4) and marine noise (MSFD Descriptor 11, D11) around both the North Sea and Celtic Seas (OSPAR, 2020). The project has provided valuable information on marine mammals and fish and uses weather information from the Met Office and the Royal Navy, satellite data from Plymouth Marine Laboratory (PML) and tidal information from NOC to inform piloting the gliders.
The ECOWind programme (2022 - 2026) has funding of ~ £7.5 million provided by The Crown Estate’s Offshore Wind Evidence and Change Programme (OWEC) and the Natural Environment Research Council (NERC) to investigate and understand how OWFs effects ecosystems and interactions between species. Furthermore, through the four key projects outlined below, ECOWind aims to enhance marine observations through innovative technology combined with numerical simulations to understand the effects of OW on marine life.
PELAgIO (part of the ECOWind programme) will explore the impacts of OW development across all levels of the food chain including physical changes to the mixing and stratification induced by structures. Relevant data will be collected via two field programmes in 2023 and 2024. Ship based and AUV observations of temperature, salinity, turbulence, chlorophyll, dissolved oxygen, fish and seabird abundance during the stratified spring-summer period. The project will develop existing models to predict impacts of stratification and mixing changes on marine mammals and seabirds.
The Fully Autonomous Marine Protected Area Seafloor Survey (Shetland ALR trial) has been commissioned, with the overarching objective to carry out a fully autonomous survey in an offshore marine protected area to demonstrate the concept and produce the data necessary to test the appropriateness of the technique to generate UK policy-relevant assessments of natural capital and ecosystems. The mission aims to demonstrate survey repeatability, water column oceanographic survey, detailed 2D seafloor mapping and general seafloor transecting survey.
Government frameworks in the North Sea
There are a number of policies and frameworks in place to monitor fisheries to support the integration of European environmental and fishery management (Jennings & Le Quesne, 2012). The main policies driving this integration are the Habitats Directive (Council Directive 92/43/EEC), the Common Fisheries Policy (Council Regulation (EC) No, 2371/2002) and more recently the MSFD (Council Directive, 2008/56/EC) (Bean et al., 2017; OSPAR, 2010).
Across the UK the monitoring of contaminant concentrations and their biological impacts in offshore marine waters is funded by Defra. At offshore locations, the long-term contaminant monitoring programmes are derived from our commitments to OSPAR Joint Assessment and Monitoring Programme (JAMP) carried out through the Co-ordinated Environmental Monitoring Programme (CEMP) (Bean et al., 2017). The UK is also a signatory to several water-related directives for the protection and maintenance of coastal and marine water quality. Various EU directives consider the assessment of eutrophication through measurement of key indicators such as concentrations of nutrients, Chl-a and DO (Devlin et al., 2011), e.g. the Urban Waste Water Directive (Council Directive, 91/271/EEC), the Nitrates Directive (Council Directive, 91/676/EEC), the Habitats Directive (Council Directive, 92/43/EEC), the Water Framework Directive (Council Directive, 2000/60/EC) and the MSFD (Council Directive, 2008/56/EC), the Oslo Paris Convention (OSPAR, 2003a, 2003b; Bean et al., 2017).
Non-Indigenous Species (NIS), also known as non-native or alien species are monitored via a number of international measures; The Regional Seas Conventions (e.g., OSPAR in relation to the UK); the EC Regulation on the use of Alien Species in Aquaculture (Council Regulation (EC) No, 708/2007); the MSFD descriptor two (Council Directive, 2008/56/EC); the Water Framework Directive; the EC Regulation on Invasive Alien Species of EC Concern (Council Regulation (EU) No, 1143/2014); and the International Maritime Organizations International Ballast Water Convention (e.g., Olenin et al., 2016; Bean et al., 2017). Although these programmes are focused on marine wildlife, they could also provide relevant data regarding water column physical and biogeochemical structure.
Industry in the North Sea
Driven by the increasing demand by the general public, government regulators and environmental organizations, the energy industry sector has had an increasing need to assess, monitor and reduce its environmental impacts. Reports on EIAs provide a useful tool in gathering and documenting environmental impact data. These assessments also assist decision makers to quantify impacts of proposed activities and plan for appropriate mitigation measures.
There are different types of environmental assessments, each having specific purpose(s) and addressing specific audience(s). In depth, environmental assessments are typically carried out on behalf of offshore energy operators by a broad range of industry survey and consultancy organisations. This assessment work is typically carried out during the site selection and pre-construction phases of an offshore site development in order to gain consents. However, there can be comparatively little ongoing environmental assessment conducted in the post-construction phases, if it is not part of a consent condition. The impact of this is that most of our understanding about the true environmental impacts of these structures, and on how they continue to affect their surrounding environments, comes from other areas including the research and academic sector.
The physical impacts of an OWF on the surrounding environment can be significant. Below are some examples of what is currently monitored as standard by industry stakeholders:
- OWF scour and sediment transport studies: Many areas around the UK coastline where OWFs have historically been built contain highly mobile sediments. Mobile sediments, coupled with the fact that many OWFs are built in water depths < 60 m means that significant quantities of sediment can become suspended in the water column and transported away. In some waters around the Scottish coastline there have already been events where some turbines have had to be removed due to extreme sediment transport altering the bathymetry of the surrounding environment. This is a phenomenon that is being studied increasingly by industry due to the cost and efficiency implications. This is typically assessed ~ yearly/every two years through bathymetry surveys. Other physical processes are thoroughly studied and investigated throughout the pre-construction phases, such as the assessment of the following parameters:
- Conductivity, temperature and density (CTD) water column profiles
- Waves – significant wave height, maximum wave height, wave period and direction
- Currents – surface and water column
However, ongoing measurement is primarily limited to current and projected physical site conditions to aid with operations and maintenance activities, such as determining if it is safe for maintenance vessels to enter the site and transfer personnel onto the structures.
Generally, the effects on the physical and biogeochemical processes are thought to be less significant for developments in deeper water, such as those sites being looked into for FLOW developments. Therefore, there is less monitoring conducted to investigate currents, scour and sediment transport in more remote offshore areas (including for O&G).
- O&G developments, associated archived data and ongoing data collection: From the beginning, it became evident that the challenging conditions in the North Sea, particularly in the northern regions, required a special focus on environmental considerations throughout the entire development process (Larminie et al., 1987). No one had previously tackled the task of developing oilfields or laying large-diameter pipelines in waters of such depth and distance from the shore. Reliable baseline data about the physical and biological environment was limited. The initial focus was on the physical environment and substantial efforts were needed to gather information on various factors, such as wind speed, wave heights (including the 100-year wave), wave amplitudes, velocities, and frequencies, sediment conditions on the seabed (pertinent for platform foundations and pipeline installation) and bottom currents. Even at this stage biological information was needed, as fouling was an important consideration for platform design and maintenance.
Concerns about the industry's impact grew. The risks to human safety were highlighted by the 1988 Piper Alpha disaster. Environmental concerns were also increasingly voiced at around the same time, particularly as the central and northern North Sea supports a large proportion of the North Sea fish catch as well as significant O&G activity. It was not until 1984, over ten years after the first production platforms were placed in position, that the first statutory obligation for environmental monitoring was put on operators to monitor the environmental impact of their activities (Declaration of the International Conference on the Protection of the North Sea, Bremen, 1984). Though there was no legal requirement for environmental studies initially, many operators conducted them to assess environmental status, particularly on the seabed in the vicinity of the platforms (Kingston et al., 1987). Initially, it was thought that the major input of oil contaminants would be in the form of oily water resulting from platform drainage and production and formation water (Department of the Environment, 1976). Predictions of broad scale areas affected by these platform discharges meant that initial studies used extensive grids of 20-30 sampling stations for monitoring impacts. However, as more information became available, particularly on the impacts of oil-based drilling cuttings, the focus shifted from the midwater to the seabed closer to platforms. Surveys tended towards transect based approaches, radiating from the platform centre.
Over five decades, over 770 O&G structures have been installed in the UK North Sea alone. The balance between the economic gain and environmental costs of this industry have led to a complex policy landscape aimed at managing licensed activities and economic growth. Understanding the industry's impacts in the region is complex due to various factors including fisheries, shipping, and eutrophication. The North Sea's ecological baseline has been lost prior to being properly documented, making it challenging to assess the scale and persistence of historical industry impacts (Henry et al., 2017). Now, many developments in the North Sea are ending their useful life and are being decommissioned, leading to new requirements for monitoring to support decommissioning plans and monitor the long terms effects of removal options (Jones et al., 2019).
iii. Existing data and potential gaps
CTD / hydrographic data including turbidity
Ocean models based on the assimilation of all available ocean observations, can provide detailed information on the 3D structure of currents in an area of operations. From the 1990s, the offshore oil industry started to use 3D numerical models to estimate impacts of environmental conditions on offshore structures for both design and operational purposes (Skliris et al., 2021). Thirty years ago, the oil industry sponsored the North European Storm Study (NESS) to undertake modelling of North European Continental Shelf wind, wave, and current conditions (Peters et al., 1993). This was followed up by another oil industry consortium, the North-West Approaches Group (NWAG), investing in measuring the current regime west of Shetland and developing a 3D ocean model (Turrell et al., 1999).
Structures and Seafloor
Offshore O&G infrastructure has long been known to be rapidly settled by attached marine life and known to support large populations of mobile animals, like fishes (Fujii, 2015) and influence feeding interactions (Fujii, 2016; Russell et al., 2014). These artificial reef complexes support diverse and productive marine communities (Claisse et al., 2014). Increasing use of imagery collected during routine structural surveys is revealing the spatial and temporal dynamics of these communities. However, there are no broad datasets available yet for the North Sea. It is clear that the biological response to installation is rapid (e.g. Todd et al., 2019; Redford et al., 2021) and changes over time (Gates et al., 2019). Structures can host species of conservation importance (Gass & Roberts, 2006) and can provide an interconnected network of hard substratum that is environmentally important at the scale of the North Sea (Thorpe, 2012; Henry et al., 2018).
Most of the early wells were drilled using diesel-based drilling fluids, as these were cheap and readily available. It became clear relatively quickly that these were having a major impact on the seabed (Hartley et al., 2003). Monitoring programmes focussed on these impacts and efforts were made to reduce the environmental impact, through switching to synthetic and eventually water-based drilling fluids.
There are generally two main approaches to most environmental studies of the seafloor carried out in the North Sea: sediment analysis and monitoring of benthic macrofauna. Obtaining reliable quantitative samples of offshore sediments with its fauna is difficult. The method employed in almost all early North Sea surveys has involved the use of some sort of seabed sampler, such as a grab or corer. For example, a 0.1 m2 area grab sampler has been commonly used to sample stations placed, 200, 500, 800, 1200, 2500 and 5000 m from the installation (Kingston, 1992). More recently, seabed imagery has provided non-destructive data (Jones et al., 2019) and other innovative approaches are also being assessed, for example environmental DNA (eDNA) sampling (Mauffrey et al., 2021).
In terms of available data, much of what is available from these sampling programmes is held in the UK Benthos Database. Currently, (v.4.06) it contains data from 11,353 stations across 351 structures including platforms, wells and manifolds, which were mapped and made available online during the North Sea Interactive project (BGS, 2014). Although the data originate from numerous separate surveys and have many issues that complicate synthesis, they have been used successfully to demonstrate that effects were limited to within 1 km from the platform and persist for at least 6-8 years in the northern and central North Sea (Henry et al., 2017).
There are a variety of other datasets that would be useful to understand the seafloor environment of the North Sea. Acoustic data on seafloor depth and hardness are particularly informative, helping to map seafloor habitats, developments and disturbances. Some of these data are collected through dedicated surveys, for example using side-scan sonar or multibeam bathymetry. Others are inferred from principally sub-surface seismic surveys. These data are compiled and presented through initiatives such as the General Bathymetric Chart of the Oceans (GEBCO).
iv. Strategic assessment
Data collection efforts have been made as part of O&G Strategic Environmental Assessments (SEAs) carried out by UK Government through the Department for Energy Security & Net Zero (DESNZ) formerly DTI, BERR, DECC and BEIS. The SEAs collected and brought together data in various areas of the UKCS (SEA areas 1-8), in addition to an SEA for Round 2 wind leasing. These were more recently combined as offshore energy SEAs (OESEA, OESEA2, OESEA3 and OESEA4) incorporated the entire UKCS (with the exception of Northern Ireland and Scottish territorial waters for renewable energy, and Scottish territorial waters for carbon dioxide transport and storage), for technologies including O&G exploration and production, gas storage and offloading including carbon dioxide transport and storage, renewable energy (including wind, wave and tidal power), and offshore hydrogen production and transport. The SEA includes amongst other things an assessment of the environmental characteristics of areas likely to be significantly affected, an assessment of any existing environmental problems and the likely significant effects of activities on the environment at a regional level. The government has maintained an active SEA research programme; identifying information gaps (some of which are outlined in previous SEA Recommendations) and commissioning new research where appropriate. This has been part of the department’s offshore SEA programme since 1999.
v. Current state of the art and adopting future technologies
Projects like e.g. ECOWind Accelerate at operational wind farms in Liverpool Bay, have demonstrated the use of autonomy for environmental data collection (AUV seafloor imaging, water column sensor and geophysical data).
The O&G industry conducts environmental monitoring in offshore areas where exploration, development, production and decommissioning activities take place. The information collected supports environmental management activities, assists in meeting regulatory requirements and provides valuable data on the state of the marine environment.
As outlined by Jones et al. (2019), hundreds of O&G structures in the marine environment are approaching decommissioning, and operations in such areas will need to be supported by environmental assessment and monitoring potentially over the life of any structure left in place. Monitoring of the general seabed environment and water column properties can incur in relevant costs for industry and the public, meaning that pragmatic and cost-effective solutions need to be considered.
Thus, traditional methods of marine monitoring, used during the development of the UK O&G industry, are now being supplemented by new, often automated monitoring techniques (Bean et al., 2017). Already available marine autonomous systems provide a wide range of solutions, allowing to collect acoustic, visual, and oceanographic data via sensors deployed on MAS. Besides the cost savings, autonomous solutions can provide a substantial improvement in the temporal and spatial resolution of environmental monitoring; however, the advances in marine autonomy require also the development of effective and efficient approaches for the purposes of monitoring.
Effective evaluation of impacts, and therefore evaluation of the optimal approach for monitoring associated with e.g. decommissioning, is aided by clear guidance on the most relevant environmental factors to consider (Table B. 1).
Unmanned, self-contained autonomous vehicles (MAS, including AUV, ROV, USV, etc.) have been used to monitor the marine environment for over a century (e.g. Ekman recording current meter; Sverdrup et al., 1942). The greatest revolution in MAS, to date, started with the Swallow float (Swallow, 1955) and led to the global ocean autonomous monitoring network ‘Argo’, an array of ~ 4000 autonomous sensor systems now surveying the upper 2000 m of the world's ocean (e.g. Medhaug et al., 2017). These ‘simple’ floats have further evolved to sophisticated particle sensing and capturing instruments (Lampitt et al., 2008), and to highly successful underwater gliders (e.g. Rudnick et al., 2004). The last two decades have seen a dramatic rise in the numbers and types of autonomous systems operating in the marine environment, and in the types of sensors these systems now carry (Wynn et al., 2014). Autonomy lends itself well to cost-effective long-term and large-scale monitoring programmes and is important in a variety of contexts (Danovaro et al., 2016).
The basin-wide decommissioning of the North Sea O&G infrastructures may be an important case to be considered also in parallel with other contexts, e.g. OWFs, where infrastructures posing, operation and decommissioning stages may have a relevant impact on the local environment. The environmental monitoring of these activities presents a challenge with the standard approaches used that can be solved for the future using MAS. Table B. 1 provides some examples of established versus potential autonomous methods for marine environmental monitoring which, for the purpose of this document, focuses on the water column.
The capability and use of autonomous/semi-autonomous devices has increased rapidly in recent years, enabling oceanographic observations at spatial and temporal scales impossible from traditional ships (Rudnick et al., 2004; Hartman et al., 2012). Autonomous vehicles are increasingly applied in marine geoscience (Wynn et al., 2014), habitat mapping (Robert et al., 2014), and benthic ecology (Morris et al., 2016), and can be divided into static, Fixed-Point Observatories (FPO; Cristini et al., 2016), and a variety of mobile platforms. The latter can be broadly split into underwater gliders, autonomous underwater vehicles (AUV), unmanned surface vehicles (USV), and seabed landing and crawling vehicles.
Existing data (e.g. samplings of the water column properties) and recommended procedures for environmental monitoring at O&G sites can be used to provide baseline information in the North Sea area and guidance for designing monitoring programmes at OWF sites.
When investigating primary oceanography data, MAS can provide a trade-off between monitoring efficiency and costs, as they greatly increase temporal and spatial resolution, they can be scoped for remote intervention in the form of adaptive surveys (depending on monitoring needs), and they represent an excellent support for satellite monitoring. However, we also need to consider disadvantages such as the limited physical sampling capability of the MAS. Note that requirements for environmental monitoring are likely to vary between environments, perceived threats, and jurisdictions. Thus, to optimise the use of this type of technology, we need to clearly define the information that should be used to establish as an environmental monitoring norm, including:
- Consider the monitoring objectives.
- Define whether the physical sample is essential.
- Directly address primary ‘Essential Ocean Variables (EOVs)’.
- Consider the spatial and temporal resolution needed for the sample collection.
Similar to the case of O&G developments, long-term monitoring of OW developments is likely to be necessary. Current standard practice does allow monitoring of the water column; however, the temporal and spatial resolution of that effort is typically limited by the high cost of ship time. MAS do offer significant potential to reduce that financial and carbon-footprint cost and reduce human risk of seagoing operations; however, its ability to offer a major uplift in the temporal and spatial resolution of environmental monitoring data should also be given serious consideration. Also, to maximise monitoring strategies considering the lifespan of an offshore project, it is important to integrate:
- Evolution of data needs throughout the lifecycle of an offshore project.
- Measurement variables and sampling techniques to address in monitoring programmes.
- Use of established methods for monitoring versus the use of autonomous systems.
- Data management and quality assurance methods to improve confidence in monitoring results and ensure the long-term usability of data.
- Use of numerical modelling in the design of monitoring programmes.
- Application of results from monitoring programmes.
Characteristic | Purpose | Established method | Established indicator | Autonomous method | Most similar indicator from autonomous approach | Compatibility of indicators |
---|---|---|---|---|---|---|
Oceanography | Primary environmental charactersation | Moored (FPO) and ship-deployed instruments | Temperature Salinity, Oxygen, pH, Turbidity, Current speed; etc. | Sensors deployed by glider, AUV or FPO | Temperature Salinity Oxygen, pH, Turbidity, Current speed; etc. | Common instrumentation |
Water quality | Monitoring contaminants | Physical sampling and sensors from surface vessel; in-situ ecotoxicology | Contaminant concentration | Sensor deployment by glider, (cruise / hover) AUV; USV, FPO; very limited sampling capacity | Contaminant concentration or proxy value | Largely sensor only; limited sampling capability |
Zooplankton | Biological effects | Physical sampling, acoustic/visual survey from surface vessel (tow-body/ROV) | Zooplankton abundance and composition | Visual and acoustic survey by (cruise/hover) AUV, FPO and USV (acoustic) | Zooplankton abundance and composition | Common instrumentation for visual and acoustic methods; limited sampling capability |
Fish | Biological effects | Physical sampling, acoustic and visual survey from surface vessel (tow-body/ROV) | Pelagic fish abundance and composition | Visual and acoustic survey by (cruise/hover) AUV, FPO and USV (acoustic) | Pelagic fish abundance and composition | Common instrumentation for acoustic and visual methods; no sampling capability |
Marine mammals | Biological effects | Visual survey; passive acoustic monitoring | Marine mammal abundance and composition | Visual survey by AUV; passive acoustic survey by AUV/USV/ FPO | Marine mammal abundance and composition | Common instrumentation for acoustic and visual methods; no sampling capability |
Contact
Email: ScotMER@gov.scot
There is a problem
Thanks for your feedback