Offshore Wind Sustained Observation Programme (OW-SOP): scoping report
Physical processes are important as they influence the productivity of the phytoplankton which form the base of the entire North Sea ecosystem. This project recommends approaches to assess the potential impact of offshore wind farms on physical processes.
3 How to predict change using models?
Chapter 1 outlines how OWFs in quiescent, less tidally energetic, regions have the potential to cause notable changes to mixing which could impact the extent, timing and magnitude of seasonal stratification. Such changes to the mixing and stratification in shelf seas like the North Sea could thus, over-time, lead to changes in the behavior of fish, seabirds and marine mammals (via 'bottom up' trophic cascades) (Figure 3.1).
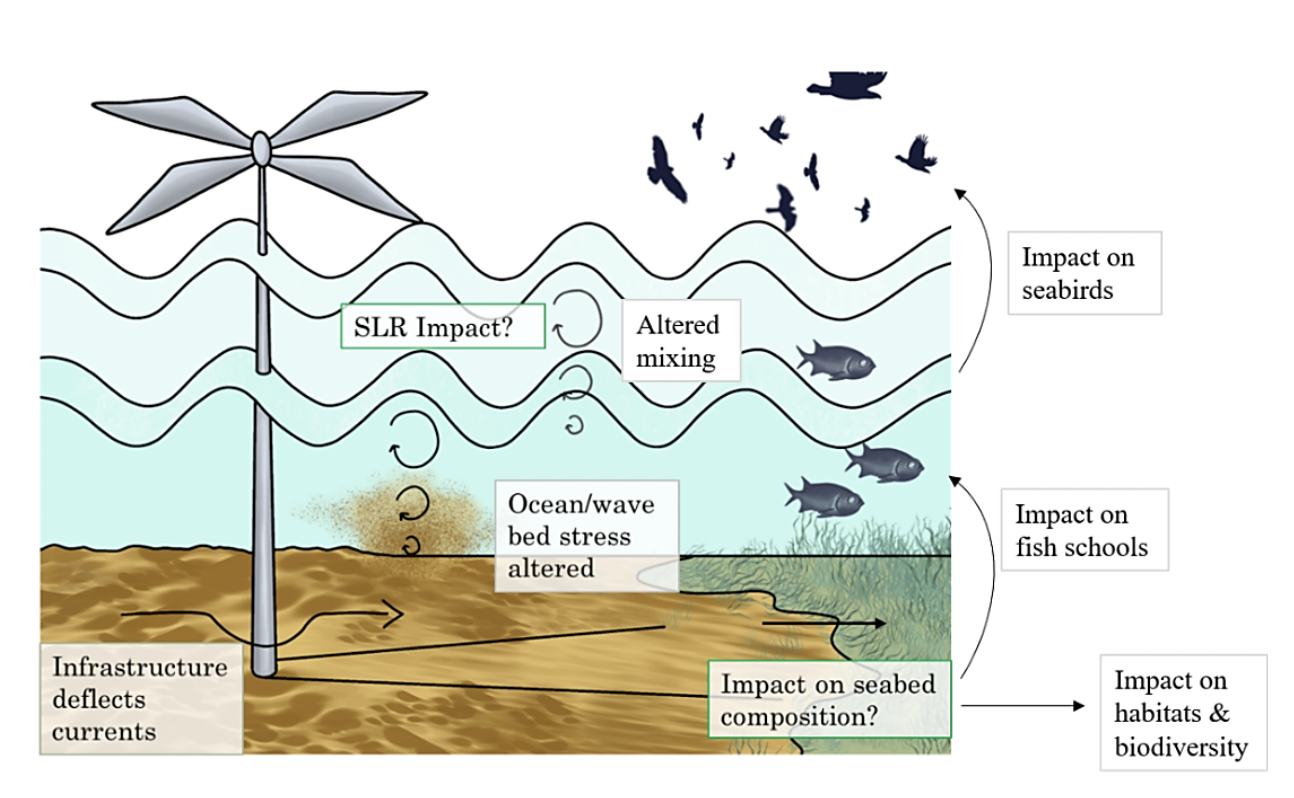
Numerical modelling is essential for predicting changes to the marine environment and marine ecosystem due to the presence and operation of offshore infrastructures such as OWFs and due to processes such as climate change, especially in absence of direct data and in support of existing data. This chapter discusses:
- Understanding impact pathways on physical and biogeochemical water column processes, also highlighting the potential impacts at higher trophic levels (Section 3.1).
- Modelling the chain of impacts: how understanding and prediction of the accumulated chain of impacts can feed into the planning and impact assessment process, and modelling tools (Section 3.2).
3.1 Understanding impact pathways on physical and biogeochemical water column processes
The mechanisms/pathways through which an OWF can change the physical water column are (Figure 3.2):
i. Induced drag of the underwater structures (fixed or floating) and mixing in their wake within OWFs, and
ii. Change of the wind field in the wake of the OWT/OWF (wind-wake effect), within and extending beyond the area of individual OWFs.
Note that, although changes to seabed conditions due to placement of infrastructures such as anchors, substructures cables, etc. on the seabed are also relevant at an impact assessment level, these types of impacts are outside the scope of this project and therefore are not discussed in this report.
3.1.1 Underwater structure effects
To date only two studies have been reported observing OWF foundation-induced mixing in occasionally stratified waters in the German Bight, North Sea. Floeter et al. (2017) performed surveys using a TRIAXUS (a remotely operated towed vehicle, ROTV), through two non-operating OWFs. This means they were able to measure the effects of the fixed underwater structures only. Water property transects through the OWF (with 80 foundations) revealed a weakening of the local summer stratification and effects extended into the surrounding area. Upwelling at the edges of the OWF was also observed. However, they concluded that it was difficult to disentangle OWF-induced changes from natural variability.
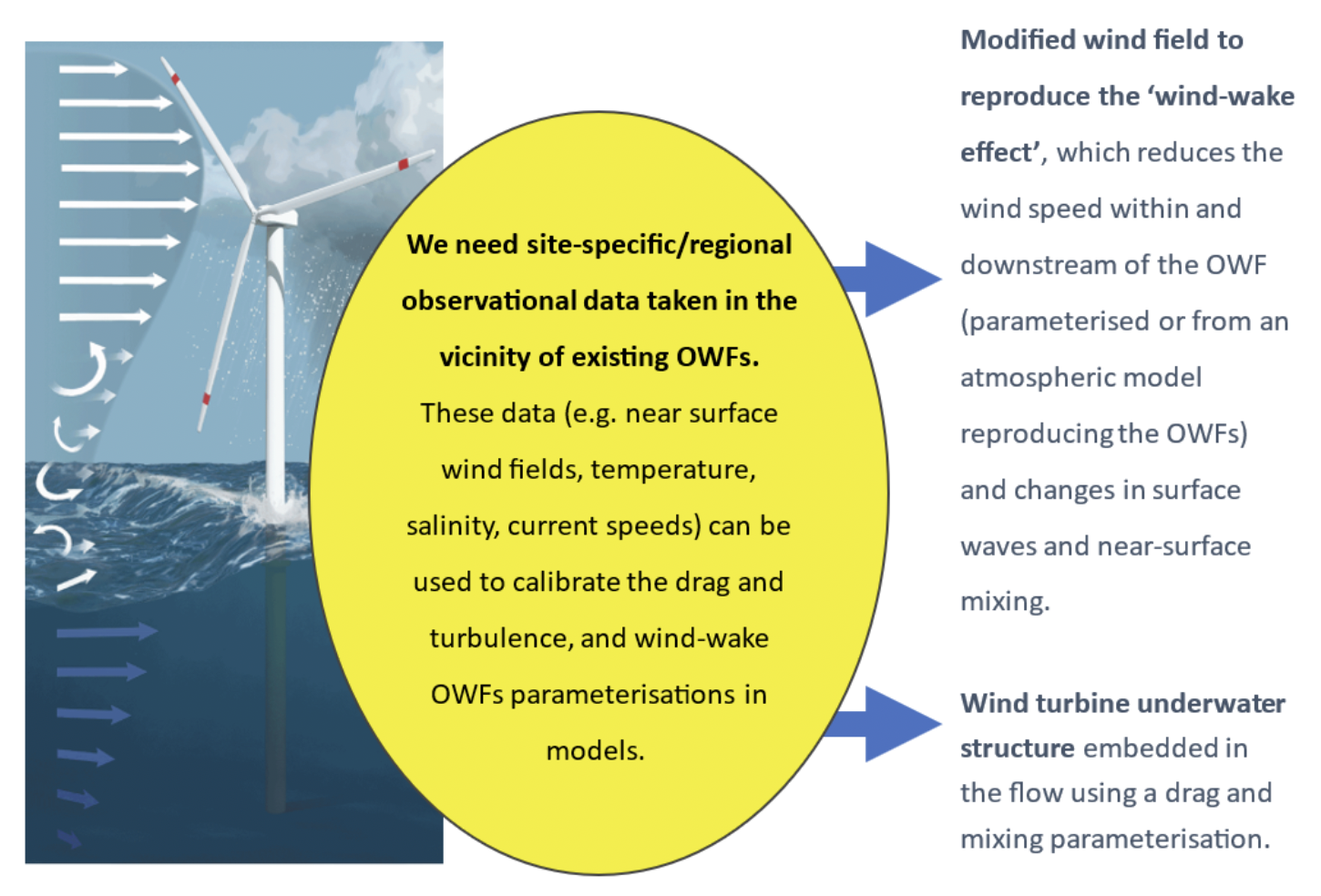
Schultze et al. (2020) measured the wake of a single monopile using a chain of CTD sensors. This was done twice in two different years. In one survey, during weak stratification, the disruption of background stratification by the wake was observed within a narrow region of up to 70 m width that reached at least 300 m downstream of the monopile. However, in a second survey, with stronger stratification, no clear signal from the monopile could be identified which stood out from the naturally occurring variability. Schultze et al. (2020) concluded that, depending on the strength of stratification, single monopiles can significantly alter the vertical structure of the water column within its wake.
Both the Floeter et al. (2017) and Schultze et al. (2020) studies were in shallow waters (< 40 m). To-date, no observations of OWF effects have been completed in deep waters, seasonal or permanently stratified shelf seas. These conditions are the subject of the PELAgIO programme, which is underway during 2024 with results still unpublished. Recent studies have addressed the potential consequences of OWFs using models. Existing models evaluating foundation impacts can be divided into two categories: models of relatively small spatial extent (1 - 2 km) that are high-resolution non-hydrostatic models (usually micro-scale Large Eddy Simulations (LES) models) with individually resolved support towers or turbines focused on effects local to turbine foundations within and close to individual OWFs; and model spanning much larger spatial extents (10s - 100s km) of lower resolution such that individual OWTs or OWFs are parametrised using (usually) sub-grid scale models and suited to capturing far-fields effects across coastal shelf regions. The surveys described in Schultze et al. (2020) were complemented by LES simulations of flow past a single monopile which was simulated under different levels of background stratification. Turbulent Kinetic Energy (TKE) dissipation rate was found to be up to two orders of magnitude larger with the monopile and persisted far downstream of the cylinder.
In this chapter we will mainly focus on the large-scale changes to physical processes potentially caused by OWFs foundations and how these may impact higher trophic levels. Structure-induced mixing has rarely been addressed at regional scales, and research is being undertaken to determine regional implications of the additional mixing generated by OWFs foundations. Since fixed OWFs foundations typically have diameters of the order of 5 - 10 m, mixing induced by structures is usually introduced as a sub-grid scale parameterization in large-scale ocean models (Rennau et al., 2012; Carpenter et al., 2016; Christiansen et al., 2023). Cazenave et al. (2016) used an unstructured grid where the wind monopiles are explicitly described in the grid, i.e. enabling representation of individual (cylindric) OWT foundations with no need for sub-grid specific parameterization of structure-induced hydrodynamics and mixing. This approach has been further tested by Christiansen et al. (2023) and compared with the sub-grid parameterization. With the grid resolving OWT foundations, Christiansen et al. (2023) encountered numerical problems due the high resolution and hydrostatic approximation. They instead recommend the sub-grid parameterization as a suitable method for assessing the large-scale impacts of structure-induced mixing.
The sub-grid parameterization of foundation drag and wake turbulence has been described in Rennau et al. (2012), Carpenter et al. (2016) and Christiansen et al. (2023). It is based on an additional quadratic friction term in the momentum equations as well as additional production terms in the dynamic equation for TKE and its dissipation rate (further details of this methodology is presented in ‘Induced drag and mixing of the underwater structures (fixed or floating)’ of Appendix C).
Review of this subject shows that no published literature is available on the combined potential impacts of fixed foundations effects and wind-wake effects (see sub-section 3.1.2). Additionally, no published literature is available on how water column changes imposed by the fixed foundations may translate into biogeochemical changes.
3.1.2 Wind-wake effects
The operational principle of an OWF is to extract kinetic energy from the atmosphere and convert it into electricity. This results in the creation of a region of reduced wind speed in the shadow zone of an OWF, the so-called wind-wake (Figure 3.2). Examples of earlier studies and new approaches investigating the oceanic response to wind stress anomalies in models are presented in ‘Wind-wake effects’ in Appendix C.
In the North Sea, an expansion of wind energy means OWFs are being developed in seasonally stratified deeper waters with lower tidal velocities, where natural tidal mixing effects are weaker and any changes in vertical transport and density distribution are more significant due to stronger stratification. In conclusion, it is not only atmospheric conditions that determine the impact of OWF wakes on the ocean, but also the regional hydrodynamic conditions in the respective environment.
Based on results of studies summarised in Appendix C, the wind-wake can generate changes in the surface mixed layer depth and dipoles in the sea surface elevation, which can affect the stratification and indicate potential impact on marine ecosystem processes. The atmospheric and the regional hydrodynamic conditions will determine or modulate the impact of the wind-wakes on the ocean. The combination of both wind-wake and underwater structure effects has not been addressed in any study; thus, it is unknown if one effect has a greater impact than the other and if they interact.
3.1.3 Physical and biogeochemical changes impacting ecosystem function via ‘bottom up’ trophic cascades.
Changes to the physical marine environment can propagate to the wider marine ecosystem. At ecosystems levels, effects of OWFs may or may not be severe, and could be positive or negative (Daewel et al., 2022). The evaluation of ecosystem effects through Before-After-Control-Impact (BACI) surveys, although possible, is challenging. The aim of the BACI method is to estimate the state of the environment before and after (BA) any change and to compare changes at reference sites (or control sites) with the actual area of impact (CI; the OWF area). Any set of ecosystem/environmental variables can be monitored within a BACI-type survey, but investigations are usually limited to abundance and diversity metrics for selected fish and sea bird species (van Berkel et al., 2020). If sampling is sufficiently representative, the measurements should, in principle, allow the disentanglement of OWF impacts from exterior regional trends. However, a number of factors make it difficult for a BACI survey to disentangle the potential impacts of OWF-induced physical and biogeochemical changes in the water column from natural variability and from regional/global trends (e.g. climate change), as well as from other concurrent impacts of other human activities like changes in fishing effort, noise levels or eutrophication (van Berkel et al., 2020).
BACI studies will also be limited by the local scale of the survey, while indirect impacts via ‘bottom up’ trophic cascades on the pelagic and benthic ecosystem can be complex and non-local. The modifications in mixing and stratification will change nutrient availability in the uppermost part of the ocean. The complexity arises from:
i. The changes in nutrients, starting a cause-effect chain that translates into changes in primary production, altering the food chain.
ii. In dynamic systems with strong tidal and residual currents, changes in nutrients are non-local because they are modulated by advective processes.
iii. Changes will be different with different hydrodynamic conditions, making it difficult to disentangle changes induced by OWFs from natural variability.
Numerical modelling studies are the only way to build BACI studies with the ability to simulate with and without OWFs scenarios, with data then required to ensure that the interdependences within and between modelling tools are fully understood to provide confidence in the predictions made. The first study to use a modelling approach to investigate ecosystem changes was van der Molen et al. (2014), which used a low resolution hydrodynamic and ecosystem model to simulate the impacts of a single OWF in a shallow well-mixed area of the North Sea (Dogger Bank). The OWF was parameterised simply as a 10% wind speed reduction only within the wind farm (and not in the wake of the wind farms). They found that the OWF would lead to a general increase in primary productivity, with the effects mostly limited to the OWF area. This is probably due to the very simplified parameterization used and to the weak currents in the area studied.
More recently, Daewel et al. (2022) demonstrated that large scale OWF developments can have a substantial impact on the structuring of coastal marine ecosystems at basin scale. The wind-wakes effects on the hydrodynamic conditions propagate at the ecosystem level. Large-scale changes in annual primary production can reach up to +/- 10% locally, and they are not only in the location of the OWFs but distributed over a wider region. Daewel et al. (2022) also found an increase in sediment carbon in deeper areas of the southern North Sea due to reduced current velocities, and decreased oxygen inside an area with already low oxygen concentration.
In summary, numerical modelling studies are the only way to build BACI studies with the ability to simulate with and without OWFs scenarios. A high-density suite of physical and biological observations data is then required to ensure that the interdependences within and between modelling tools are fully understood to provide confidence in the predictions made. There are no studies available on the impacts of the underwater structure of OWFs on the ecosystem functioning. Daewel et al. (2022) is the only study addressing how and to what extent atmospheric changes generated by large OWF clusters could affect marine productivity. However, there are no studies available on how those impacts could further propagate in the food chain.
3.2 Modelling the chain of impacts: how it can feed into the planning and impact assessment process, and modelling tools
Modelling tools are essential to explore potential pathways and impacts of OWF developments, allowing marine spatial planners and developers to understand the complex environmental impacts of any new installation. The aim of modelling studies can be to reduce uncertainty in cumulative impact assessments. Models can cover the continuous range of processes from physics through chemistry, to ocean productivity, prey and predators. This allows assessment of the potential cumulative impact on marine physics and biology in the water column and at the seabed. Typical modelling tools used for these impact assessments are hydrodynamic and wave models such as NEMO, FVCOM, TELEMAC, SWAN, WaveWatchIII, and TOMOWAC (Figure 3.3). They can give information about changing currents, waves, seawater characteristics (salinity, temperature) and mixing/stratification. The physical models must then be coupled with biological processes models to inform ecosystem models such as ERSEM, and particle tracking models, to give information about the ecosystem functioning and productivity (Figure 3.3).
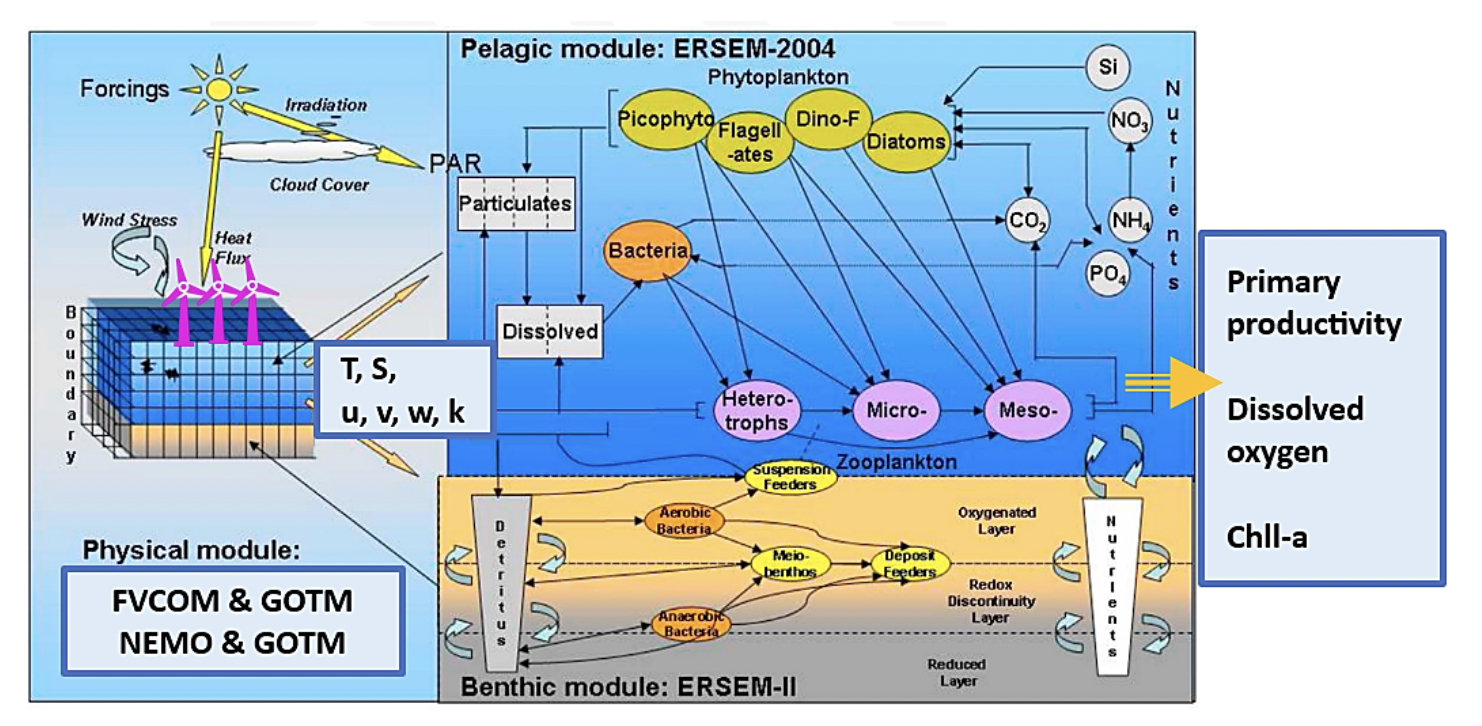
3.2.1 Models enable investigation of local and non-local effects of OWFs
While essential for planning, local (in-situ) observations at OWF sites can only present part of the picture. Numerical models can be used to investigate both near-field and far-field impacts, and to cover the range of scales from device-scale to far-field effects in the wider regional seas and spanning a range of timescales. The choice of modelling tools must be fit for the scale, e.g. computational fluid dynamics code for device scale, unstructured models to represent turbines, shelf-scale models to understand far-field impacts of OWFs.
3.2.2 Models enable investigation of future scenarios
Using numerical tools, we can explore both present and future impacts, and ‘what-if’ future scenarios. This can be the placement and layout of new OWFs, as well as the interaction between turbines and the marine environment in a changing climate. Models can also suitably address different time scales, to understand both immediate and long-term environmental impacts. With deterministic simulations, we can understand the chain of processes that alter the environment and may identify surprising and unanticipated consequences of OWF installation. This ‘future knowledge’ would be impossible with observations alone, while at the same time observations are required to validate models before using them in a predictive mode. These exploratory scenarios use model realisations and storylines to engage stakeholders in conversations and to understand and manage competing needs.
Ocean and biogeochemical model predictions could then become a very powerful tool at the planning stages to help determine the size and locations for future OWFs in order to minimise the perturbations on the natural environment. Ocean simulations could also be used to understand the integrated and cumulative impacts with regard to the interactions between several individual OWFs. Understanding of the integrated and cumulative impacts can be used to optimise the utilisation of the offshore space, making sure that future OWFs are laid out in a way that minimises cumulative effects on the environment. However, as the number of turbines grows, so does the uncertainty related to their combined effect. This is especially true of emergent fields e.g. FLOW, where little data exists.
3.2.3 Models are essential to disentangle potential OWF effects from natural variability, climate change and other external pressures
Any set of ecosystem/environmental variables can be monitored within a BACI-type survey, however, a number of factors make it difficult for a BACI survey to disentangle the potential impacts of OWF-induced physical and biogeochemical changes in the water column from the natural variability and from the regional/global trends (e.g. climate change), as well as from other concurrent impacts of other human activities like changes in fishing effort, noise levels or eutrophication (van Berkel et al., 2020). Other than high-density spatial and temporal physical and biological observations, numerical modelling studies are the only way to build BACI studies with the ability to simulate with and without OWFs scenarios.
Ocean simulations are essential for understanding the relevance of the environmental effects. Any OWF installation will perturb the environment, and defining which is the acceptable limit of change is a difficult task for decision makers. Models could help improve understanding if the environmental effects observed are within the natural variability, i.e. if they are within the natural fluctuations of the ocean system. Climate change will also perturb the system (beyond its natural variability) and understanding if the modelled environmental effects of OWFs are larger or smaller than climate change effects is also important. OWFs could also interact with climate change effects either to exacerbate, or to mitigate. As stated in Dorrell et al. (2022), the introduction of wind turbine structures into deeper stratified water will provide a new, artificial, source of turbulence (Section 3.1). This increased turbulence could potentially offset the impacts of climate change on stratification and increase the supply of nutrients to the surface layer and oxygen to the deep water. Careful wind turbine design and layout (guided by models) could therefore potentially provide an important tool in mitigating impacts from climate change.
Some examples of ‘ongoing modelling efforts’ are summarised in Appendix C.
Contact
Email: ScotMER@gov.scot
There is a problem
Thanks for your feedback