Offshore Wind Sustained Observation Programme (OW-SOP): scoping report
Physical processes are important as they influence the productivity of the phytoplankton which form the base of the entire North Sea ecosystem. This project recommends approaches to assess the potential impact of offshore wind farms on physical processes.
4 Where and when to observe change - sustained observation programmes
There are only three observational studies that detected water column changes associated with submerged OWTs and surface wind-wake effects, with several modelling studies that have started to investigate both effects (Dorrell et al., 2022; Carpenter et al., 2016) (Figure 4.1). More observational studies are therefore needed to support modelling approaches, to develop and validate parametrisations and to validate simulation results.
Some indication of the potential effects of OWF infrastructure at the current construction levels is emerging which suggest that multiple wind farms could have a cumulative impact at a regional scale (Christiansen et al., 2023).
A total of 867 findings on pressures due to wind energy devices and impacts on ecosystem elements were extracted from 158 publications as part of this project. Half of the analysed publications (51%) presented empirical evidence, while 36% of the studies were based on modelling approaches, including the modelled propagation of underwater noise. Literature reviews accounted for 11% of the publications, and only 1% of the studies were based on expert judgement. A continuous increase in the number of publications was identified, especially in the last eight years (74% of the scientific publications), which is in line with the increase of OWFs and installed production capacity.
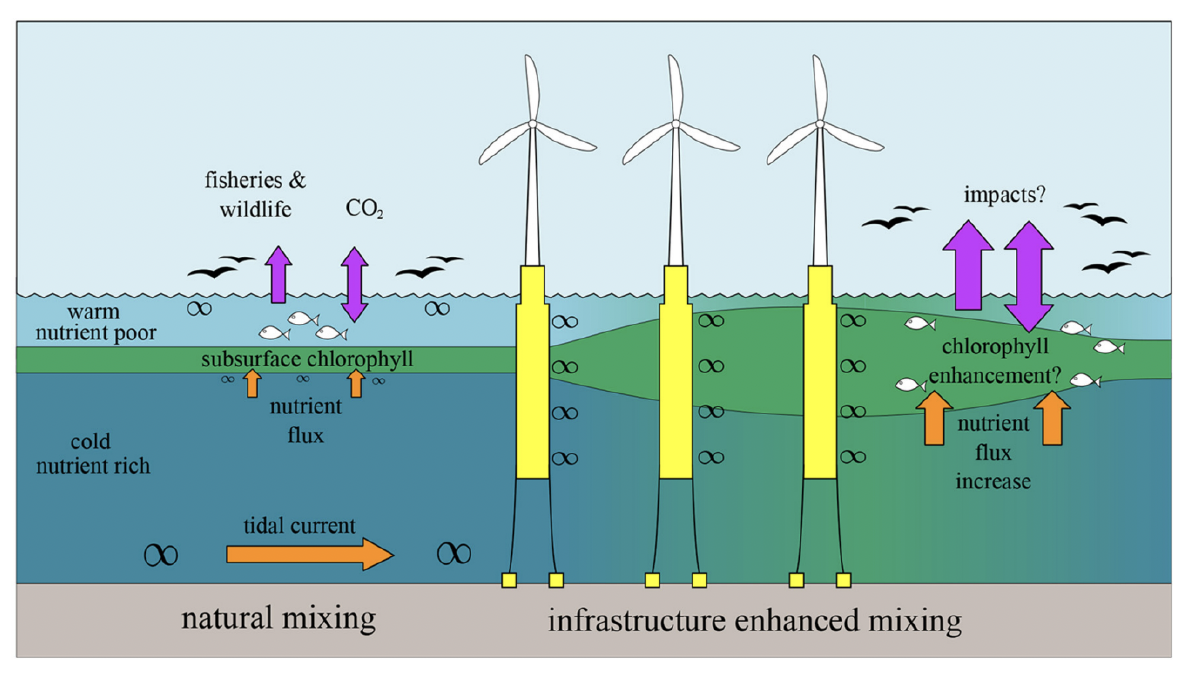
Studies have been conducted in shallow seas (North Sea, 66% of the publications), during the operational phase (64%), in shallow waters (90% at < 30 m depth), close to the coast (56% < 20 km offshore), with few turbines (80% with < 81 turbines), low production capacity (63% with < 160 MW), and a small area (67% < 70 km2).
There is a general shortfall in the coordinated prioritisation of issues to be addressed by observational and monitoring programmes. Much of this lack of prioritisation stems from an incomplete evidence base across all aspects of the potential impacts of OWFs, which has hampered the objective selection of priority topics. However, the quantity and breadth of peer-reviewed evidence continues to accumulate and has now allowed some to conduct meta-analyses and identify which impacts are of greatest concern (Galparsoro et al., 2022), as well as the remaining thematic gaps within the current evidence base.
Amongst the theme of observational programmes, this chapter focuses on:
- Gaps in existing observational/monitoring programmes (Section 4.1).
- Priority variables and monitoring techniques for impacts directly or indirectly mediated by water column processes and pelagic biodiversity (Section 4.2).
4.1 Gaps in existing observations/monitoring programmes
A comprehensive analysis of the gaps associated with water column observational / monitoring efforts within OWFs can be split into the following groups:
i. Gaps and failings associated with the design, execution, interpretation and reporting of monitoring activities (sub-section 4.1.1).
ii. Gaps associated with the observation of complex interactions within a heavily utilised ecosystem (sub-section 4.1.2).
iii. Gaps associated with specific components or processes of the ecosystem, and consequently, the collection of specific abiotic and biotic variables (sub-section 4.1.3).
4.1.1 Gaps associated with the design, execution, interpretation and reporting of monitoring activities
The cost of monitoring activities will always constrain the spatial and temporal extent of monitoring programmes. Most compliance-based monitoring programmes are often impact-driven (rather than cause-effects) and locally specific (Bean et al., 2017). Equally, most monitoring programmes are configured for the detection of short-term, high-magnitude changes (Lindeboom et al., 2011). It is widely recognised that longer-term changes are poorly covered (Lindeboom et al., 2015). This is a particular weakness when considering the likely duration of some of the processes of interest due to OWFs, and due to climate change. Spatially and temporally limited observations hamper our ability to scale issues in areas (e.g. from turbine to farm-to-farm cluster or capturing spatially offset far-field impacts) and over time (e.g. understanding change associated with long trajectory processes). This impact-driven approach has not always enabled areas of high model uncertainty to be reduced.
Any monitoring programme must present uncertainty and confidence estimates alongside their output. Ideally, methods for calculating uncertainty would be standardised across monitoring efforts. Equally, the required level of uncertainty needed for a specific regulatory aspect or management action should also be stated (Franco et al., 2015); the combination of an uncertainty value with a specific threshold of quality for a management action derives a level of confidence.
Ensuring that monitoring methodologies are fit for purpose at the point of commissioning requires minimum detectable change (MDC) or effect sizes to be specified and associated with target variables i.e. what magnitude of change needs to be detected within the programme (Franco et al., 2015; Lindeboom et al., 2015). Specifying these values ensures there is enough replication within the monitoring programme to reliably report on change concerning the MDC. However, specifying MDC values is complicated by the limited understanding about the natural variability of the baseline and how relevant deviations from this baseline are. Equally, the level of heterogeneity within monitored areas is often inadequately accounted for, thereby increasing the apparent natural variability within datasets, and consequently reducing the ability to reliably detect change at a specific level.
4.1.2 Gaps associated with the observation of complex interactions within a heavily utilised ecosystem.
Across the collective monitoring effort, some programmes need to be tasked to consider both negative and positive changes of high magnitude. The need to reliably observe negative change is obvious. Changes seen to be positive also require dedicated monitoring effort. Recording positive change is necessary so that indirect or trophic cascades are documented and considered during holistic assessments. Furthermore, the use of compensation as a management tool for achieving no net loss (Hooper et al., 2021) / balancing net gain within an area will also rely on the accurate estimation of both negative and positive changes associated with specific human activities or structures. Finally, the monitoring of positive changes should also be sustained over a substantial time scale so that the longevity of the benefits can be assessed and how benefits will also scale both spatially and temporally.
Although an old issue, the inherent complexity represented by multiple trophic levels, pressures from a single human activity and multiple human activities remains daunting. As such, other than simplistic additive approaches (e.g. Halpern et al., 2008 - for multiple pressures and activities), there remains a lack of evidence that prevents the constriction of tools to accurately represent these complexities within inherently complex ecosystems in a heavily utilised environment (Lindeboom et al., 2015). Long-term processes, such as climate change and ecosystem change following the cessation of fishing are high priorities for merging with more acute changes. However, as there are few opportunities to observe these trajectories now, it makes their integration in cumulative impact assessments particularly difficult (ICES, 2020). There is a lack of a ‘total ecosystem’ approach (Bean et al., 2017). Observations are not currently integrated into a modelling system, and this limits the development of consistent understanding of cause-effect, transferability, future change, cascading and indirect effects, and cumulative impacts. The total ecosystem approach is described as a “coherent evidence-to-advice package” that relies, at its heart, on “a dynamic model of the ecosystem function and its responses to pressures based on process relationships” (Bean, et al., 2017). Rather than interpreting monitoring data directly, the information is used to refine the parameterization of the model. The modelled ecosystem response could then be used to interpret change rather than the monitoring data directly. There are many benefits to this approach which include:
- Specific datasets update the relations of many variables and processes within the model;
- Feedback can be given to the value of the new information added to the model, thereby supporting adaptive approaches to marine monitoring; and
- Modelled outputs can be reported in a quantitative manner using specific units of monitoring, management or scientific value.
4.1.3 Gaps associated with specific components or processes of the ecosystem, and consequently, the collection of specific abiotic and biotic variables
Having discussed some of the broader gaps on how modelling programmes are designed (i.e. move from ‘local impact driven’ to ‘drive refinement of the model framework’), the following section will look at specific processes and the individual variables best used to monitor them. Variables will be flagged when these are not collected routinely, using an appropriate method for informing on processes within OWFs or through existing programmes. Table 4.1 highlights the following processes, and associated variables, that are only partially covered by existing efforts and are therefore inadequately observed for providing informative cause-effect relationships or robust monitoring conclusions. Topics and variables represented in Table 4.1 focus particularly on hydrodynamic conditions associated with water column mixing. Sentinel variables are:
- Profiles of current speed and direction, turbulence and suspended load.
- Profiles of physical-chemical properties of seawater (i.e. temperature, salinity, dissolved gas, nutrients, chlorophyll and suspended particulate matter).
Descriptor: Hydrodynamic conditions associated with water column mixing. | ||||||||
---|---|---|---|---|---|---|---|---|
Sentinel variables | Sentinel priority | Possible methodologies | Specific information value of method | Cross-descriptor value | Ease and reliability | Active monitoring in place | Cost | Topic total |
Profiles of current speed and direction, turbulence and suspended load | Very high | Monitoring using ship mounted ADCP | 2 | 1 | 3 | 2 | 1 | 9 |
Monitoring using AUV mounted ADCP | 3 | 1 | 2 | 2 | 2 | 9 | ||
Monitoring using ADCP on mooring or seabed lander | 3 | 1 | 2 | 2 | 2 | 9 | ||
Profiles of physical-chemical properties of seawater | High / Very high | Monitoring using CTD profiling and water sampling from a ship | 2 | 1 | 3 | 1 | 1 | 8 |
Monitoring using CTD profiling from an AUV | 3 | 2 | 2 | 1 | 2 | 9 | ||
Monitoring using a CTD sting on a mooring | 3 | 2 | 2 | 1 | 2 | 9 | ||
Monitoring using remote sensing (satellite imagery) | 2 | 2 | 1 | 1 | 2 | 9 |
4.2 Priority variables and monitoring techniques for impacts directly or indirectly mediated by water column processes and pelagic biodiversity
Galparsoro et al. (2022) summarised priority monitoring gaps, weighted by the methodology used, suggesting the following ranking:
High priority (Table 4.1):
- Hydrodynamic conditions associated with water column mixing.
Medium priority (not considered further in this report)
- Biodiversity of benthic habitats influenced by water column processes.
Low priority (not considered further in this report)
- Seafloor integrity (i.e. the loss and gain of natural and artificial substrata, and also included as turbine surfaces transect the water column).
This report focuses on the high-priority topics associated with changes in water column properties, although it recognises that water column mixing can have consequences on the biodiversity of pelagic and benthic habitats as well as fish aggregation and recovery post-fishing. These high-priority topics were rated as being important as they represented a significant source of uncertainty within the holistic understanding of potential OWF impacts. Equally, the existing monitoring activities provided by statutory sampling programmes, industrial surveys/compliance monitoring (all sectors) and scientific investigations reveal little existing coverage of this topic in terms of spatial or thematic alignment.
Using a coarse ranking of monitoring methods for examining water column properties suggested that in-situ or the use of MAS is likely to be the most cost-effective monitoring technique long term. These monitoring methods also scored highly on their ability to provide either high spatial extent and/or temporal resolution. Remote sensing techniques for identifying turbidity plumes downstream of turbines are also an established technique for providing proxies of mixing. However, site-specific conditions can mean that a surface turbidity plume, indicative of water column mixing, is not always present (van Lancker & Baeye, 2015). Equally, the availability of cloud-free days around the UK limits the reliability of this technique as a monitoring tool (Kumar et al., 2021).
With regard to plankton sampling, both ship-based sampling and moored, in-situ instrumentation scored highly (Galparsoro et al., 2022). Although the ship-based techniques are expensive and lack the temporal resolution provided by in-situ instrumentation, they provide the ability to undertake a range of monitoring techniques (e.g. ADCP and CTD profiling) and thereby improve the cost-effectiveness of a multiparameter monitoring cruise. Crucially, ship-based monitoring allows the collection of water and plankton samples for analysis in the laboratory, yielding measurements of biogeochemical parameters (with low uncertainly) unobtainable from MAS. Concerning stock assessment work within the zone of influence of OWFs, scientific trawling within the footprint of OWF may not be possible. This means traditional scientific trawling may be restricted to the edge of the OWFs, which provides a more ambiguous assessment of fish aggregation, recovery and potential spill over. Alternatively, vessels using scientific acoustic fish finders that do not deploy fishing gear may be more likely to be able to access OWFs. Although less quantitative, this monitoring method also benefits from being able to be undertaken from smaller, cheaper vessels (or even MAS).
Contact
Email: ScotMER@gov.scot
There is a problem
Thanks for your feedback